Type in a location above, or select from the list below.
Zipcars live in 500+ cities across North America and Europe. See all Zipcar cities
Detroit, MI
Honolulu, HI
Houston, TX
Los Angeles
Minneapolis, MN
Milwaukee, WI
New York/New Jersey
Philadelphia
Sacramento, CA
San Diego, CA
San Francisco Bay Area
Washington DC
Golden Horseshoe
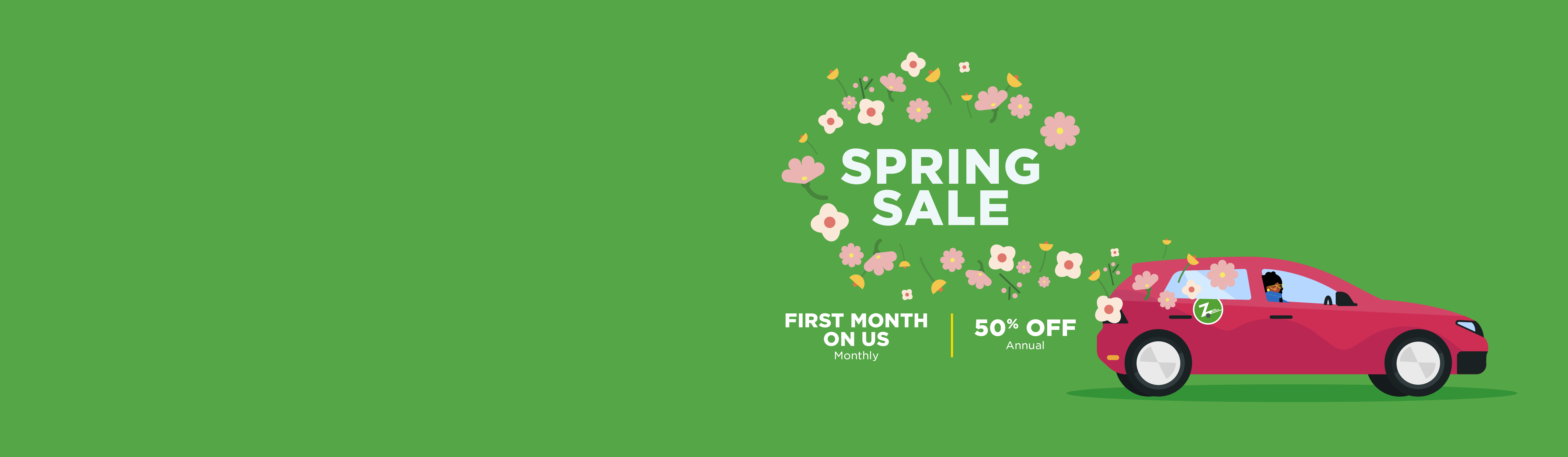

Instantly book cars near you
Book on demand by the hour or day, with gas and dedicated parking included.
For a limited time, get 50% OFF an annual plan** or your first month on us* with code: SPRING.
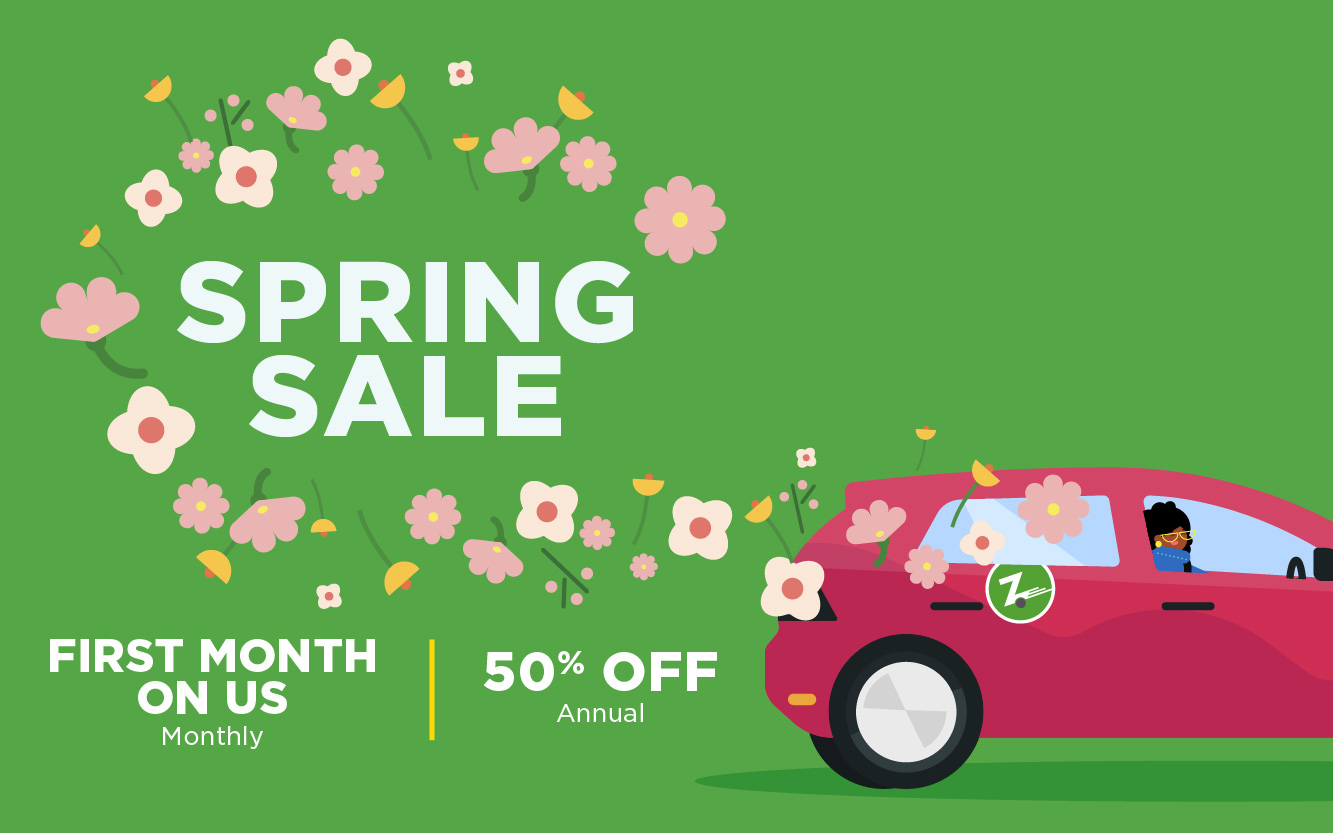
How Zipcar works
Join in minutes.
Apply online with a valid driver's license. Most people are approved instantly and can book a trip within minutes.
Drive on demand
Book a round trip car by the hour or day. Use our car sharing app to unlock and lock during your trip.
When you're done, park the car in the same location you picked it up from, then end the trip with the app.
Homepage Find Cars Full Width Map
Zipcar in boston, zip north shore or south shore. go outlet shopping at wrentham. or just cruise around your favorite square—davis or copley explore more with the world's leading car-sharing network., zcpc car carousel, meet some of our cars, zipcars come in all shapes and sizes—compact sedans, luxury suvs, vans, and more. switch it up whenever you feel like it., why zipcar, reserve wheels when you want them, by the hour or day, and only pay for the time you drive..
Zipcar covers gas, insurance options,* parking, and maintenance for a potential savings of $1000/month over car ownership.
Cars near you
Zipcars live in your local neighborhood, and in cities, campuses and airports across the globe.
Drive on-demand
No waiting in line at the counter. (No need to even search for your keys!) Just book and go.
Go beyond public transit
Zipcar is the perfect complement to the bus and train—whether it’s local errands or weekend adventures.
Cars around Campus
Get off campus and save with your student discount
Find my school
Cars for business
Simplify your transportation with low weekday rates and experts on your side.
Ready to drive?
The backseat isn’t an option where you’re headed. it’s time to start commuting, exploring, and running errands on your own time., frequently asked questions.
More Questions?
* Offer valid for new Zipcar members’ first month of membership only. Application fee still applies. Excludes current Zipcar members. Zipcar may change or revoke the discount any time. Membership Discount excludes Annual, Business and University rate plans, will be applied to Monthly membership rate plan only, and has no cash value.
**Offer valid for new Zipcar members’ first year of membership only. Excludes current Zipcar members. Offer expires 6/24/2024. Zipcar may change or revoke the discount any time. Discount applied to annual membership rate plan only and has no cash value. Application fee still applies. Membership Discount excludes Business and University rate plans.
Starting rates are estimates only and do not reflect variations due to discounts, availability, holidays, or other factors. Actual prices may vary. Other fees may apply . You may review your total estimated reservation cost before you confirm your reservation.
A non-refundable $25 application fee applies when you submit your Zipcar application.
- ©2024 Zipcar, Inc. All rights reserved.
- Terms of Use

- Recruitment Privacy Notice
- Accessibility
- United States (EN)
- Canada (EN)
- United Kingdom (EN)
It looks like you might be in the . Would you like to update your location?
Unlock cars 24/7 with your phone, and go!
Car rental by locals
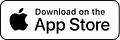
Choose from thousands of cars available from private and professional owners near you.

Trip liability insurance is included. You can even add another driver at no extra cost.

Book a car near you instantly, even at the last minute. No lines. No paperwork.
Our secure Getaround Connect technology allows you to do the walkaround inspection of the car with the app. The car opens. The keys are inside. Off you go!
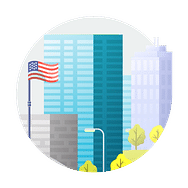
Looking for a car in the US?
Switch to our US website
Own a car? Put it to work!
Wondering how to get around Paris during the Games? Learn more about control perimeters
Coming to Paris? We've gathered all the information you need to know about the Opening Ceremony
San Francisco offers many cultural & historical tours. Let's see why it is so famous for visitors!
Rent the perfect vehicle with Getaround car rental services There are a thousand reasons why you may need a rental car. Maybe you’re moving and need a cargo van to move your furniture across town. Perhaps you’re going on a romantic getaway with your partner and want to rent a convertible to cruise around in. Or maybe you’re going on a business trip and need a luxury rental car to impress your clients! No matter what your needs are, Getaround is the perfect car rental option.
Getaround car sharing is an environmentally friendly , convenient, and affordable alternative to traditional car rental. When you rent a car with Getaround, you’re actively choosing to share an individual owner’s car when they wouldn’t be driving it anyway. That helps keep new cars off the road, giving the environment a tiny break it desperately needs.
Getaround cars are conveniently located across communities, at airports, and in cities around the world. That means car-sharing guests benefit from having affordable and convenient access to nearby rental cars. But there’s more! Getaround car rental is super flexible. You can rent a car by the hour or by the day. And, if your plans change, no big deal! You can cancel within 1 hour of booking or more than 24 hours before the start of your trip for free.
- Download the free Getaround app to your smartphone. Search for the vehicle you’d like to rent based on location and duration (by the hour or day…), and book the vehicle that best meets your needs.
- Start driving! The Getaround app turns your smartphone into a car key. You can unlock the car directly from the app, meaning you have full flexibility to get the car any time after your rental pickup time.
Getaround offers a huge array of fleet styles including trucks, vans, cars, convertibles, SUVs, and so much more. The variety of sizes, styles, colors, models, and makes is as large as the community or area where you’re renting a car. When you search for a car to book on the Getaround app or website, you can filter by your needs.
In our category filter, you can choose the type of vehicle you need: SUV/Jeep? Coupe/Sedan? Minivan? Pickup truck? The car rental options are endless. In our class filter, you can choose between economy, luxury, and performance. And guess what? You can even filter by transmission (automatic or manual) and by drivetrain (all-wheel drive, anyone?). But it doesn’t end there! You can even filter by special features. If you’re going on a ski trip and need a roof rack, you can search specifically for that. Pet-friendly cars, cars with bike racks, and cars with Bluetooth… You can request whatever you need from your Getaround rental car.
Whether you’re /going on a road trip with friends or taking a weekend getaway, Getaround car rental meets all your vehicle rental needs.

Enter your search term
Search by title or post keyword
What is Carsharing – AKA “Airbnb for Cars”?
8 best carsharing services, the car share rental process, frequently asked questions, take control behind the wheel, airbnb for cars: 8 best carsharing services [& how they work] in 2024.

Since 2012, Brett Helling has built expertise in the rideshare and delivery sectors, working with major platforms like Uber, Lyft, and DoorDash.
He acquired Ridester.com in 2014, the first ridesharing marketplace, leveraging his direct experience to enhance the site. His insights at Ridester are recognized by Forbes, Vice, and CNBC.
Expanding his reach, Brett founded Gigworker.com and authored “ Gigworker: Independent Work and the State of the Gig Economy “, demonstrating his comprehensive knowledge of the gig economy.
More about Brett | How we publish content
How Turo Go Works For Renters And Vehicle Hosts
How jayride works to offer affordable airport rides, via app: the affordable, eco-friendly solution to commuting.
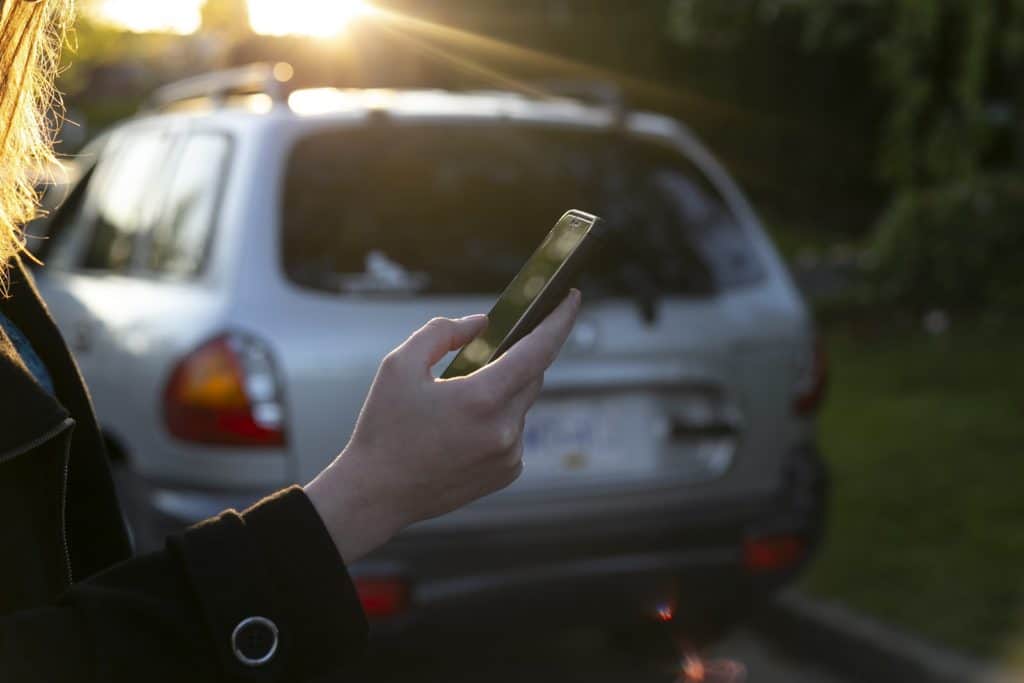
Key Takeaways
- Carsharing offers on-demand vehicle rentals, similar to Airbnb’s lodging service.
- Unlike traditional rentals, carsharing provides flexibility with app-based, self-service booking.
- Turo, Zipcar, Getaround, Maven, Enterprise CarShare, SHARE NOW, GIG Car Share, and Evo Car Share are popular services.
- Carsharing process includes booking through an app, making payments, picking up the car, and driving.
Carsharing, often referred to as the “Airbnb for Cars,” is a service that allows individuals to rent vehicles on-demand through app-based, self-serve systems.
It provides a convenient way to rent cars by the hour, minute, or mile, with vehicles listed by local owners aiming to earn passive income.
This service caters to a broad audience, including urban residents, young travelers, and those seeking sustainable transportation options.
Unlike traditional car rentals, carsharing offers unparalleled flexibility, allowing users to rent a vehicle at any time without being restricted by business hours or fixed rental periods, paying only for the time or distance they use.
Below, we’ll list eight of the top car sharing services in the United States.
- Enterprise CarShare
- GIG Car Share
- Evo Car Share
Double-check your insurance before renting!
Many insurance providers exclude carsharing services as part of your standard auto policy.
Before you rent a vehicle with a carsharing service, make sure that you understand what is covered – and not covered – with your standard insurance policy.
If you’re in doubt, get the extra coverage from the carsharing company.
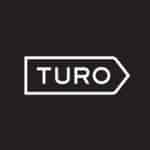
- Peer-to-peer car rental platform
- Offers a wide range of vehicles
- Insurance and unlimited mileage included
Often dubbed the “Airbnb for cars”, Turo is a peer-to-peer car rental platform that bridges car owners with individuals seeking to rent vehicles for short periods.
Unlike traditional car rental services, Turo offers a diverse range of vehicles from everyday models to high-end luxury cars and vintage classics. It’s available in various cities across the U.S., Canada, the UK, and other select international locations.
Pricing is set by individual car owners, and the cost typically includes insurance and unlimited mileage . An added benefit of Turo is the ability to deliver the car to a chosen location in some instances.
Booking a vehicle on Turo is simple. Browse the range of available cars, select your desired pick-up and drop-off dates, and message the car owner to arrange the details.
Of all the carsharing services, Turo is my personal favorite.
Turo is my personal favorite among all of the carsharing services on this list. The prices are affordable, and the cars are incredibly high quality.
For example, I recently took a trip to Waco, Texas to visit a friend. I rented a 2018 Mercedes S-class on Turo – for the same price that a 2022 Kia Forte would have been at enterprise. Talk about an upgrade!
For those looking to make some extra income by renting out their car , Turo offers an avenue to list their vehicle for rent when it’s not in use.
Download Turo on iPhone or Android .
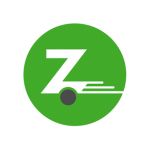
- Known car share service, global availability
- Includes gas, insurance, and roadside assistance
- Subscription-based with hourly/daily rates
Zipcar is perhaps the best-known car share service.
Available in cities and universities across the United States and around the world, the company allows users to book nearby cars 24/7 straight from its website or mobile app. You only need a reservation and a “Zipcard” that allows you to unlock your selected car.
On the Zipcar platform, you can find all sorts of cars to fit your needs, including standard sedans and luxury SUVs.
Rental rates for these vehicles vary by city (they usually start at about $9-$10 per hour) but always include gas, insurance, and roadside assistance at all hours. You’ll get 180 miles included in your reservation each day and only pay 45 cents per additional mile.
Before you use Zipcar’s services, you need to subscribe to the service for $7 per month or $70 per year (students at partnered universities may get cheaper rates). However, this gives you the flexibility to book any time and from anywhere Zipcars are available — which includes over 400 cities in the U.S. alone.
Download ZipCar on iPhone or Android .
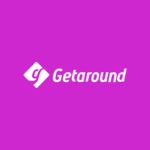
3. Getaround
- Peer-to-peer car share with competitive rates
- No membership card needed; unlock with smartphone
- Includes insurance and roadside maintenance
If you’re looking for competitive rates or a wider variety of cars, Getaround may be the service for you.
As a peer-to-peer car share, this mobile app can connect you to privately owned cars in your area, which means you’ll have far more choices when it comes to rates and vehicle models. For example, you may find anything from sedans and convertibles to pick-up trucks and cargo vans.
With Getaround, you don’t need a membership card. You can use your smartphone to locate and unlock any Getaround vehicle in your area and start a trip. Your hourly rental rates will vary widely based on the exact listing you select, and you can always expect to pay a booking fee (at least $2 and usually under 10% of your trip cost).
An “under-25” fee will apply to each trip younger drivers book. Twenty miles per hour (or 200 miles per day) are included in the initial booking cost, after which you’ll be charged 50 cents per mile or $5 per mile for specialty cars.
You need to fill up on gas at the end of your trip, but insurance and roadside maintenance are included.
Getaround allows users to book on-demand in seven countries with no annual fees. The company offers car share services in 12 U.S. states and Washington, D.C.
Download Getaround on iPhone or Android .
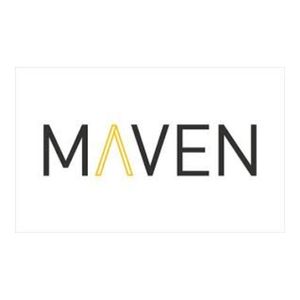
- GM-owned, offers gas, hybrid, and electric vehicles
- Operates in select U.S. cities
- No monthly or annual fees
Owned by General Motors, Maven is a safe and secure car-sharing service that allows you to rent gas, hybrid, and electric vehicles.
Much like Getaround, it enables you to find and unlock privately-owned vehicles with just your smartphone. Additionally, every sedan or SUV you drive goes through a proper safety inspection before it’s listed.
Maven has scaled back its operations quite a bit in the past year, so it’s only available in parts of Michigan (Ann Arbor and Detroit) and California (Los Angeles and San Francisco).
Drivers in these states can access vehicles on-demand with rates starting at $8 per hour with no monthly or annual membership fee.
Download Maven on iPhone or Android .
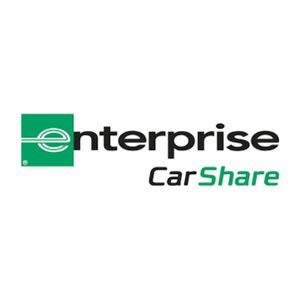
5. Enterprise CarShare
- Enterprise's car share option with a wide fleet range
- Membership card access; includes gas and insurance
- Rates vary widely by location
Enterprise is best known as a traditional car rental company but recently joined the car share space as well. Enterprise CarShare connects drivers to its trusted fleet of newer, well-maintained cars, which range from electric sedans and fuel-efficient SUVs to cargo vans and luxury cars.
Much like Zipcar members, Enterprise CarShare users both unlock their vehicles and end trips by scanning their membership cards.
Enterprise CarShare rates vary extremely widely by location. Monthly membership fees in the United States range from $5-$50, and hourly rates can start at $5-$10. Mileage rates can be 25-45 cents per mile, and you may get 200 miles free.
Generally speaking, these fees are fairly economical compared to other apps, especially considering they include gas, 24/7 customer service, and even physical damage protection.
This car share brand is most prominent in the United Kingdom, but it is available in nine cities across eight U.S. states as well as at plenty of universities and businesses in these markets and beyond. It’s also available in select Canadian locations.
Download Enterprise CarShare on iPhone or Android .
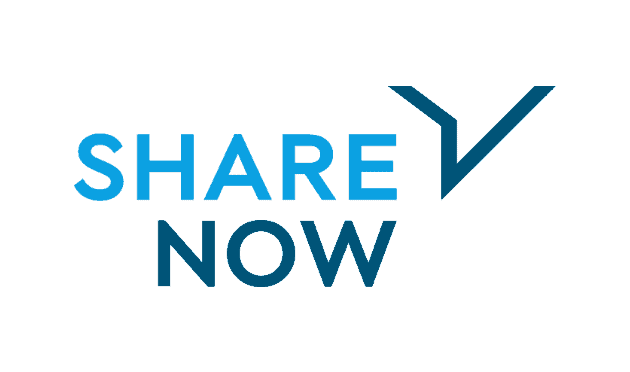
6. SHARE NOW
- Merger of Car2Go and DriveNow
- Free-floating model, drop off at on-street parking
- Minute, hourly, and daily rates; includes insurance
The result of a large merger between Daimler AG’s Car2Go and BMW Group’s DriveNow, SHARE NOW is a car sharing network that spans eight European countries and select U.S. cities.
SHARE NOW functions much like a scooter-share service with free-floating vehicles that can be dropped off at legal on-street parking spots in the operating area.
They list slightly different parking rules for each country (such as when parking is and isn’t at your own cost), so check for the restrictions in the country you’d like to use SHARE NOW.
Dropping the car off at streetside parking or parking garages makes it perfect for both one-way and roundtrip commutes.
Another unique feature of SHARE NOW is its assortment of cars. You can access models from reliable and highly coveted brands like Mercedes-Benz, BMW, MINI, and Smart at any time of the day. Some European cities currently have all-electric fleets.
With SHARE NOW, you can even rent by the minute. Rates start at 24 cents per minute and $15.99 per hour, though you can book by the day at a $69.99 flat rate.
Mileage does affect your price, but you will receive free parking, gas, and insurance with your purchase. SHARE NOW is completely free to join and currently offers a $10 credit for signing up.
In the United States, SHARE NOW is located in New York, Seattle, and Washington, D.C.
Download SHARE NOW on iPhone or Android .
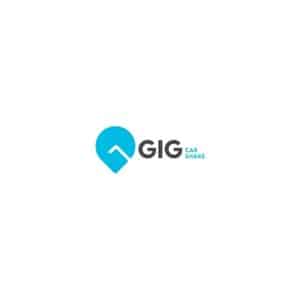
7. GIG Car Share
- AAA-powered, focuses on hybrid and electric vehicles
- Free-floating model with included gas, insurance, parking
- Rates set at per minute or hourly basis
Another option we can’t miss is GIG Car Share , an environmentally friendly car sharing service powered by the American Automobile Association (AAA).
Currently located in Sacramento and soon expanding to Seattle, the company tries to help you reduce your carbon footprint by connecting you to hybrid and electric vehicles as well as making a largely car-free lifestyle possible.
GIG Car Share works a lot like SHARE NOW, with free-floating vehicles that can be picked up and dropped off just about anywhere in your market.
Cars are unlocked via smartphone, but you can also request a membership card if you want back-up. That way, you won’t be stuck if you have spotty service).
Gas, insurance, and parking are all included in the company’s rates, which are currently set at 15 cents per minute (up to $15) and $15 per hour for both hybrids and electrics. AAA members can get discounts on rides.
Download GIG Car Share on iPhone or Android .
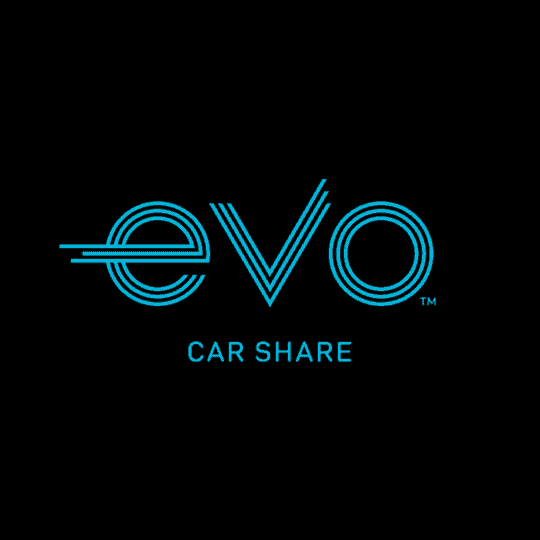
8. Evo Car Share
Based out of Vancouver, Canada, Evo Car Share offers a fleet of hybrid Toyota Prius C vehicles for environmentally conscious urban commuters.
Evo stands out with its free-floating model, allowing users to pick up and drop off vehicles anywhere within a designated home zone. This makes it especially handy for those spontaneous trips or one-way commutes.
Each Evo vehicle is equipped with bike and ski racks, appealing to the active lifestyle of many Vancouver residents. Rates are competitive, and pricing is transparent with no hidden fees. The service includes fuel, insurance, and parking in designated areas.
Evo’s focus on sustainability and convenience has made it a preferred choice for many Vancouverites looking for a green and flexible transportation option.
- Based in Vancouver with a fleet of hybrid Toyota Prius
- Free-floating model, vehicles equipped with bike/ski racks
- Includes fuel, insurance, and designated parking
While some of the details may differ depending on the different companies you choose, all carsharing companies operate along the same broad lines. Here is how the process generally works:
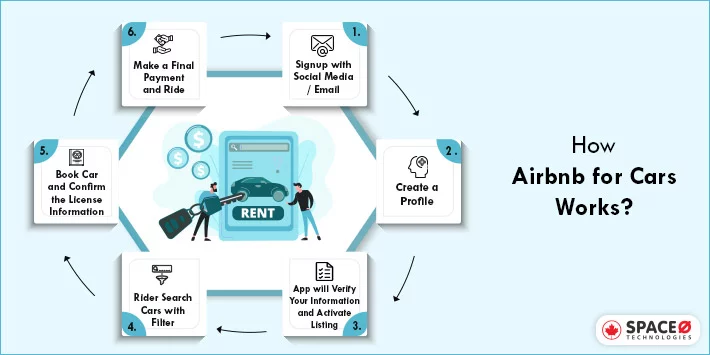
One of the primary appeals of carsharing is its seamless booking system. Users can easily browse through a variety of vehicles, selecting their preferred car, duration, and time. This is made even more straightforward through dedicated apps, allowing for hassle-free reservations at the touch of a button.
Costs will vary based on the service provider, the type of vehicle chosen, and the rental duration. Payments are typically made upfront using a credit card. It’s essential to be aware that additional fees might be incurred, such as cleaning charges for any spills or messes.
A standout feature of carsharing is the ease of picking up your chosen vehicle. Unlike traditional rentals, carsharing platforms enable users to quickly locate and unlock vehicles in their vicinity through the app. Detailed instructions guide users through the unlocking process, ensuring a smooth start to their journey.
Here’s where the adventure begins! Enjoy the freedom of driving around, whether it’s for a quick errand or a leisurely drive.
While most carsharing experiences are straightforward, it’s crucial to be aware of specific rules some companies might have – for instance, Getaround’s 200-mile limit .
Ensure you’re familiar with these to avoid any unexpected charges. Once you’re done, simply return the car to the designated drop-off point.
Read our answers to the FAQs below to learn more about car shares and how they work.
1. Can car shares be used to drive for Uber or Lyft?
Car shares are typically for personal use, making them unsuitable for rideshare drivers, especially with company logos violating Uber and Lyft standards. However, Maven Gig and Getaround’s partnership with Uber offer rideshare-approved rental options for drivers.
2. How old do I need to be to use car share services?
Car share services have varying age requirements, like Zipcar’s 21-year minimum (18 for affiliated students). Always review terms before signing up to avoid issues.
3. Is car sharing always the cheapest option?
Car shares are cost-effective for infrequent users, but regular or long-distance drivers might find long-term rentals or ownership more economical.
When you don’t have access to your own car, it may seem like taxis, rideshare apps, and public transportation are your only options. However, car shares continue to expand, providing an alternative to daily car rentals and letting you control when you stop and go — no need to wait for a driver.
With car sharing, you’ll only pay for the time you really need without taking on major recurring costs like auto insurance and car payments. If you do find the need or budget for a car, take a look at our guide to the nine best car-buying apps so you can shop wisely for your permanent ride.
1 thought on “Airbnb For Cars: 8 Best Carsharing Services [& How They Work] In 2024”
Brett; nice summary here. I am wondering why you don’t mention Turo? I thought they were the largest peer-to-peer car-sharing platform?
Leave a Comment Cancel reply
Save my name, email, and website in this browser for the next time I comment.
Related Posts
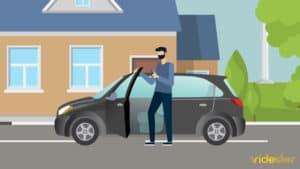
Browse our Resources
Whether you're a customer or a driver, we've made it easy to find information about how to make the most of rideshare, delivery, and transportation companies.
New to #ridesharing and #delivery? Start here!

Get all the best information about the Delivery Industry.
Explore Delivery Service companies and jobs.
Income & Payments
Find the best advice on using delivery services as a customer.
Gig Economy Knowledge from Experts
What Is Car Sharing and How Does It Work?
- University of Ottawa
GoodLifeStudio / Getty Images
- Public Transportation
Car sharing is rapidly growing in popularity, but many people still aren't quite sure what it is, how it works, and how it compares to other methods of transportation. How expensive is it? Do you have to pay for gas? What if there's no car when I need one? What about insurance? Where do you park it when you're done? Is it really better for the environment? Does it save you money? Is it available in my area? These are all questions that we're going to answer today.
Car Sharing vs. Traditional Car Rental
The first thing you need to know is that car sharing is a type of car rental. What makes it different from traditional car rental (Hertz, Enterprise, etc) is that it is designed to be convenient for people who want to rent cars for short periods of times (a few hours) and only pay for their usage (you are billed based on how long you have a car and the distance traveled).
Another difference with a traditional car rental that makes car sharing more practical for people who don't own a car is that it allows you to access a car at any hour, not just business hours. And because the cars are spread around town in reserved parking, chances are there's one such parking close to where you live, making it easy to walk to it.
Image: Zipcar
Whether you will save money with car sharing is highly dependent on your usage. For some people car sharing will be the cheapest option, for others, it will be car rental, and for others, it will be owning a car. Zipcar , a big North-American car sharing company, has some online tools that can help you estimate how much money you could save by using car sharing .
How It Works
So how does car sharing work in practice? It's pretty simple, really: First, you need to figure out which car sharing operator(s) operates where you live if any. The easiest way is probably to just do a Google search for "car sharing" plus the name of where you live. If you are in a big city, your chances are pretty good. If you are in a rural area, you're probably out of luck. Another way to find a shared ride is to look at Wikipedia's list of car sharing operators by country.
Once you have found a car sharing service to join, you need to determine what conditions they put on membership. If we look at the biggest operator in North-America, Zipcar, we see that they require the member to be at least 21 years old and to have a valid driver's license (they run a driving record check, so if you have a history of reckless driving, you might not be able to get in). If you meet the requirements, it's as simple as filling out the online registration form and picking a rate plan (for example, here in Ottawa VRTUCAR has three different plans ).
Once you are in, all you need to know is where the cars are parked, and how to reserve one. There will be a little variation from one car sharing service to the other, but most of the big ones will send you a membership car that can be used to unlock the cars. Locating them is simple: Go to your car sharing's website and there should obviously-titled section (something like "find cars") that shows you a map of your area with the locations of all the reserved parkings. Once you know which is closest, you can reserve a car either online or by phone -- that's how you know that there will be a car for you when you get there. If your car sharing service offer more than one model, you'll be able to choose (a pickup to haul lots of stuff, a Prius for normal driving, etc).
Once you get to the car, you usually unlock it with your membership card (the magic of RFID ) and that's it. As long as you bring it back to the same parking spot in one piece, that should be all. Gas and insurance are covered in what you pay (membership fee + whatever extra you pay per hour and/or mile driven). Wasn't that simple?
Is It Greener Than Car Ownership?
Is car sharing better for the environment than owning a car? And if so, how much? In the majority of cases where car sharing makes sense, it is indeed greener than owning a car. Depending on the location, each shared car can replace between 6 and 20 cars, and most shared fleets are recent models with the latest emission control, and many fuel-efficient options are usually available (lots of Prius hybrids, Civic hybrids, Yaris compacts, etc). So that's a good start: Fewer cars need to be manufactured, and the car sharing fleets are usually mostly composed of vehicles that are top tier when it comes to fuel economy and tailpipe emissions. Also, plug-in hybrids and electric vehicles will soon be part of car sharing fleets
Another way that car sharing is green is by giving strong incentives to drive less. When you own a car, you already have very high fixed costs (car payments, insurance, maintenance, parking), so driving a bit more doesn't cost you that much more than what you are already paying. But with car sharing, the relation between what you pay and how much you drive is a lot more linear. Drive half as much, and you'll pay almost half as much (your yearly membership is fixed cost). This means that a lot fewer unnecessary trips are taken, and car sharing service members tend to walk and bike a lot more because they don't feel like "well, I have a car in the driveway and I'm paying through the nose for it, I might as well use it."
The Future of Car Sharing
The world is rapidly urbanizing and in the future, most of humanity will live in cities. At the same time, billions of people will want more personal mobility. If we do things right, our cities will be designed in ways that make them walkable and bikeable, and fast and efficient public transportation will provide the majority of trips.
Car sharing can complement these means of transportation. It works better in densely populated areas (ie. cities), it works better for people who have other ways to get around most of the time (ie. for the daily commute), and it is less expensive than owning a car, especially in a city. It looks like the future is bright for car sharing!
- How to Go Green: Commuting
- 2021 in Review: The E-Bike Revolution Hits the Streets
- Should I Buy an Electric Bicycle? Here's Everything You Need to Know to Get Started!
- The Unexpected Pros and Cons of Electric Cars: Your Go-To Guide Before Buying
- Converting Your Gas-Powered Car to Electric
- What an Hour of Gardening Does to Your Body
- Does Hybrid Work Reduce Carbon Footprint? It's Complicated
- Best Electric Bike (EBike) Insurance Companies
- How to Be a Sustainable Traveler: 18 Tips
- 22 Sustainable Swaps to Make at Home
- Urban Design After the Pandemic
- How Can I Live Rent-Free?
- The Future of Main Street, Post-Pandemic
- The Seven Stones of Simpler Living
- 9 Ways to Resist the Siren Call of Consumerism
- Best of Green Awards 2021: Sustainable Travel
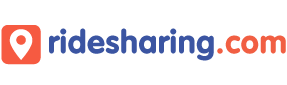
WE CONNECT DRIVERS & PASSENGERS
You are a driver? Post a ride
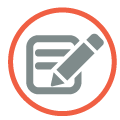
Sign up for free
Drivers and passengers donât pay any registration or membership fee
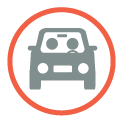
Daily commute
Find a carpool buddy for your daily commute at no cost
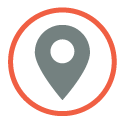
Long distance rides
Post or book a ride everywhere in Canada and the United States
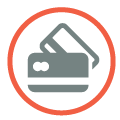
Online payment
Book and pay your seat online for long distance rides
Come aboard with 241 920 members

Forgot your password?
Become a driver
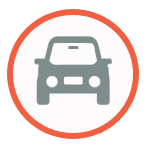
Become a passenger
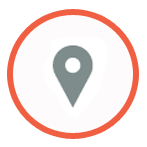
Satisfied members!
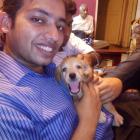
Discover the latest ride offers
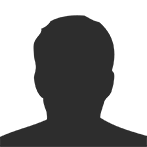
Los Angeles , CA ➜ Fremont, CA
Austin , TX ➜ Nashville, TN
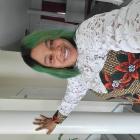
Newmarket , ON ➜ Barrie, ON
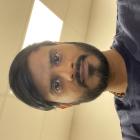
Burlington , ON ➜ Brampton, ON
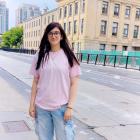
Ottawa , ON ➜ Richmond Hill, ON
Richmond Hill , ON ➜ Ottawa, ON
Brampton , ON ➜ Burlington, ON
Kelowna , BC ➜ Middlebury, IN
How works Ridesharing.com
Long distance ride share.
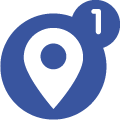
Posted ride offers
Driver posts a ride offer for a trip anywhere in Canada and in the US.
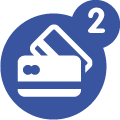
Seat booking & payment
Passenger books and pays its seat online. Driver & passenger then communicate by phone or email.
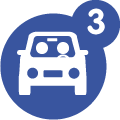
Driver & passenger meet for the rideshare. During the ride, the passenger gives the confirmation code.
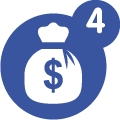
Money transfer
Driver confirms the ride with the code and cashes the money via direct deposit in its bank account , by Interac or Paypal.
Local carpool
Driver posts its offer for a local ride anywhere in Canada or in the US.
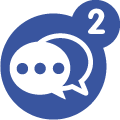
Communication
Passenger contacts the driver to request a seat for a ride and to reach an agreement (money or alternating driving).
Local carpool & daily commute
Driver and passenger meet at the meeting place to carpool together!
The Blog on Ridesharing.com
Recent posts.
You are a member of Blablacar in Europe? Sign up on Ridesharing.com in Canada and the USA
Craigslist rideshare, Facebook group & Kijiji rideshare vs the benefits of Ridesharing.com
Kangaride vs Ridesharing.com discover the benefits
Allo-Stop is closed, use Ridesharing.com today!
Discover new destinations!
Vancouver edmonton, toronto ottawa, ottawa toronto, calgary vancouver, vancouver montreal, toronto kingston, winnipeg montreal, charlottetown montreal, ottawa montreal, toronto montreal, saint-john bathurst, montreal halifax.
- Search for a ride
- How does it work?
- My seat bookings
- Cancellation policy
- Post a ride
- My passengers' list
- Ridesharing.com
- Corporate solutions
- Events solutions
- Our corporate clients
- Terms of use & privacy policy
- Legality & insurance

- Français English
- Country / Currency:
- Canada / $CAD USA / $USD
© 2005-2024 Ridesharing.com | All rights reserved
Browser not supported
This probably isn't the experience you were expecting. Internet Explorer isn't supported on Uber.com. Try switching to a different browser to view our site.
The ride options on this page are a sample of Uber’s products, and some might not be available where you use the Uber app. If you check your city’s web page or look in the app, you’ll see what rides you can request.
UberX Share
With UberX Share, get everything you love about UberX for a more affordable price— save up to 20% when matched with a rider along your route. See terms.*
Why go with UberX Share
With UberX Share you can save up to 20% when matched with another rider along your route. See terms.*
Stay on schedule
UberX Share is designed to add no more than 8 minutes to your trip on average.
Be climate smart
Help your city avoid extra emissions and car travel by sharing your ride.
Designed to help keep you moving
Request one seat only.
You can request one seat only with UberX Share. If you're riding with a friend or a group of friends, consider requesting UberX or UberXL.
Updating our front seat policy
You will no longer be required to sit in the back seat. However, to give your driver some space, we ask that you use the front seat only if you need it.
Be a 5-star rider
Our guidelines ask you to treat drivers and co-riders with respect, follow the law, and help keep one another safe. Not following any of our Community Guidelines may result in the loss of access to your Uber accounts.
How UberX Share works
Open the Uber app, enter your destination, and scroll down to the UberX Share ride option.
The app will try to match your car with other riders heading your way. Get additional savings if you’re matched with a co-rider. See terms.*
If your trip was 5 stars, consider saying thanks and tipping your driver in the app after your trip.
Frequently asked questions
You can only request one seat on UberX Share. If you're riding with a friend or a group of friends, please request UberX or UberXL.
At any given point during your trip, you may be sharing the trip with up to two other co-riders. If a co-rider is dropped off before you, it’s possible the app will search for another co-rider, but it’s designed to only look for people going your way.
UberX Share is designed to add no more than 8 minutes to your trip (note: because of traffic and other possible delays, we cannot guarantee arrival times). You’ll always see your estimated arrival time in the app as you ride.
Pickup and dropoff order is determined by where your destination falls along the route, rather than the order of who was picked up first.
The app matches you with riders going your way to help make sure any additional pickups won’t increase your estimated arrival time.
It depends on the space in the car and the number of riders. If you’re traveling with luggage, we recommend requesting an UberX ride instead of an UberX Share ride.
UberX Share is currently live in 34 metropolitan areas in the United States:
Ann Arbor, Atlanta, Austin, Baltimore-Maryland, Bloomington (IN), Boston, Chicago, Connecticut, Dallas, Denver, Gainesville, Honolulu, Houston, Indianapolis, Las Vegas, Los Angeles, Madison, Miami, Nashville, New Jersey, New Orleans, New York City, NYC Suburbs, Orange County (CA), Orlando, Philadelphia, Phoenix, Pittsburgh, Portland, San Diego, San Francisco, State College (PA), Tampa Bay, Washington DC.
UberX Share is available in 34 cities in the US and 60 cities globally. To see if UberX Share is available in your city, open the Uber app to request a ride.
Correct, Uber Pool is now UberX Share where you'll save up to 20% if you're matched with a co-rider along your way.
More from Uber
Go in the ride you want.
Sustainable rides in electric vehicles
Pick a car. See the price. Get moving.
Uber Reserve
Book a ride in advance
As many stops as you need in one car
Affordable rides, all to yourself
UberX Saver
Wait to save. Limited availability
Local taxi cabs at the tap of a button
Uber Intercity
Go city to city
On-demand electric bikes that allow you to go further
Electric scooters to help you get around your city
Auto rickshaws at the tap of a button
Affordable, convenient motorcycle rides

Uber Transit
Real-time public transit information in the Uber app
Affordable rides for groups up to 6
Uber Comfort
Newer cars with extra legroom
Premium rides in luxury cars
Uber Black SUV
Premium rides for 6 in luxury SUVs
Ride assistance for seniors and people with disabilities
Sign up to ride
Read our community guidelines.
The material provided on this web page is intended for informational purposes only and may not be applicable in your country, region, or city. It is subject to change and may be updated without notice.
Everyone using the Uber platform is required to comply with applicable laws and regulations while doing so.
*Matching with a co-rider is dependent on time of day, traffic, the number of ride requests, and the number of drivers available in a given area. Riders who use UberX Share can receive a minimum discount, and may receive more based on the time and distance traveled with other co-riders. See the app for details.
- Our offerings
- How Uber works
- Diversity, equity, and inclusion
- Sustainability
- LaGuardia Airport
- Newark Airport
- LAX Airport
- Chicago O'Hare Airport
- San Francisco Airport
- Boston Airport
- Harry Reid Airport
- DFW Airport
- Miami Airport
- Atlanta Airport
Car rentals
- Car rentals New York City
- Car rentals Chicago
- Car rentals Los Angeles
- Car rentals Las Vegas
- Car rentals Philadelphia
- Car rentals Houston
- Car rentals Boston
- Car rentals Miami
- Car rentals San Francisco
- Car rentals Washington
Car service cities
- Car service New York City
- Car service Chicago
- Car service Los Angeles
- Car service Las Vegas
- Car service Philadelphia
- Car service Houston
- Car service Boston
- Car service Miami
- Car service San Francisco
- Car service Washington
Courier services
- Courier services New York City
- Courier services Chicago
- Courier services Los Angeles
- Courier services Las Vegas
- Courier services Philadelphia
- Courier services Houston
- Courier services Boston
- Courier services Miami
- Courier services San Francisco
- Courier services Washington
Popular routes
- LAS to Las Vegas NV
- Manhattan NY to Queens NY
- LAX to Los Angeles CA
- SFO to San Francisco CA
- ORD to Chicago IL
- MCO to Orlando FL
- BOS to Boston MA
- ATL to Atlanta GA
- Miami FL to Miami Beach FL
- DCA to Washington DC
- Taxi New York City
- Taxi Chicago
- Taxi Los Angeles
- Taxi Las Vegas
- Taxi Philadelphia
- Taxi Houston
- Taxi Boston
- Taxi San Francisco
- Taxi Washington
- Investor relations
Sign up to drive & deliver
Create a rider account, order delivery with uber eats, sign up for uber for business, sign in to drive & deliver, sign in to ride, sign in to order delivery with uber eats, sign in to your uber for business account, drive & deliver, ride with uber, uber for business, manage account.
- Affiliate links
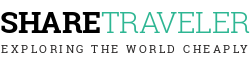
Home » Transportation » Car Rentals » Car Sharing for Travel
Car Sharing for Travel
I have updated my spreadsheet of sharing economy transportation resources and this is a summary of my finding so far. While I’m a big fan of public transportation, some places in the world it’s better, easier, and nicer to explore with a car. And fortunately for travelers, there are a wealth of new options for getting around using sharing economy resources. These fall into four distinct categories:
- Peer to peer car rentals
- Membership based car rentals
- Peer to peer taxi services
- Peer to peer ride sharing
Peer to peer car rentals expand car rental options beyond the traditional rental companies, and generally have at least slightly better pricing with more diversity of vehicles and pick up locations. If you are a car owner this is a fine way to earn some extra cash when you’re not using the car. If you are in need of a car for a day or more, either in your home town or on the road, this is a good option to consider, especially if you find rental car companies are too expensive in your location. For now this is only available in cities, but services are expanding quickly in the U.S. and Europe. For one example, see my review of Flightcar .
Membership based car rentals are generally more appropriate for people to use in their home city, but they have the added bonus of being usable on the road if the company you join has services in a city you are visiting. So if you are a member of Zipcar in San Francisco you could also use this service on a trip to Boston. These services are most cost effective when you need a car for a few hours. If you want to use a car for multiple days and/or drive more than a few miles, you’re probably better off renting a car from a commercial rental agency or from a peer to peer rental service.
Peer to peer taxi services are smart phone driven alternatives to commercial taxis, currently found in major U.S. cities. They are particularly useful when you are somewhere that doesn’t have taxis driving by. According to several recent analyses of cost across various cities, the prices of these services aren’t actually very different from using a regular taxi, especially during rush hour. Though Sidecar comes out consistently about 20% cheaper than a cab.
Peer to peer ride sharing is the option I’m most excited about for travel. This is catching on in Europe, Australia, and the U.S. Drivers join a website where they post upcoming trips they are taking and how many open seats they have, along with a price per seat. Then riders can sign up to join those trips. Drivers and riders get reviewed, and in some cases you can select traveling companions based on features like how talkative they are. I’m looking forward to trying out Blablacar or Carpooling on my upcoming trip to Spain as a way to visit areas outside of the cities where I’ll be staying. It appears that at least for some places this is cheaper than taking the train or bus. And it sounds like a pleasant way to meet locals and travel in comfort without all the unnecessary stops inherent to public transportation.
Not included in the categories above but another interesting development, there is now a ride sharing of taxi service in NYC so if you find yourself there you might want to check out Bandwagon .
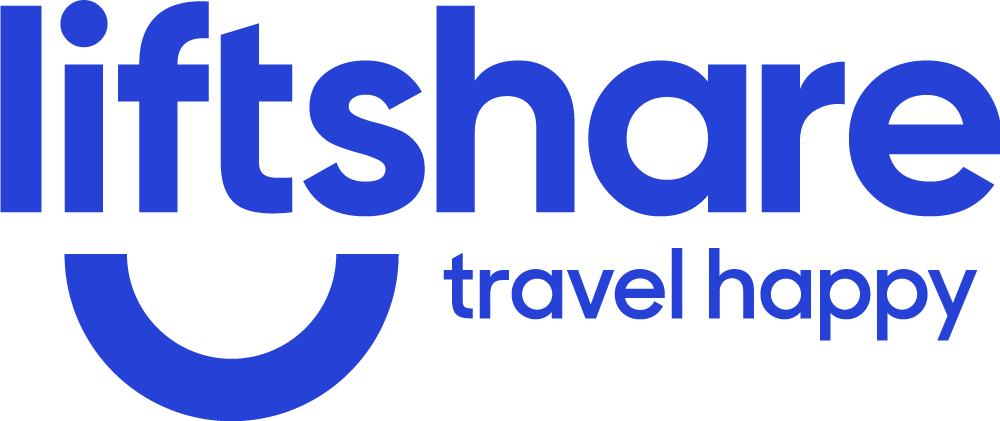
Join 700,000 like-minded people saving money and the planet by car sharing
Are you an employer.
How much can Liftshare save your organisation? try our calculator...
JOIN THE CAR SHARING REVOLUTION.
It’s the easy, fun way to cut the cost of driving.
and find both drivers or passengers to share with
Be part of the bigger picture and help to reduce CO2 emissions
Save money; our members save over £1,000 on average every year
Reduce the stress of driving. Share a lift and share the load
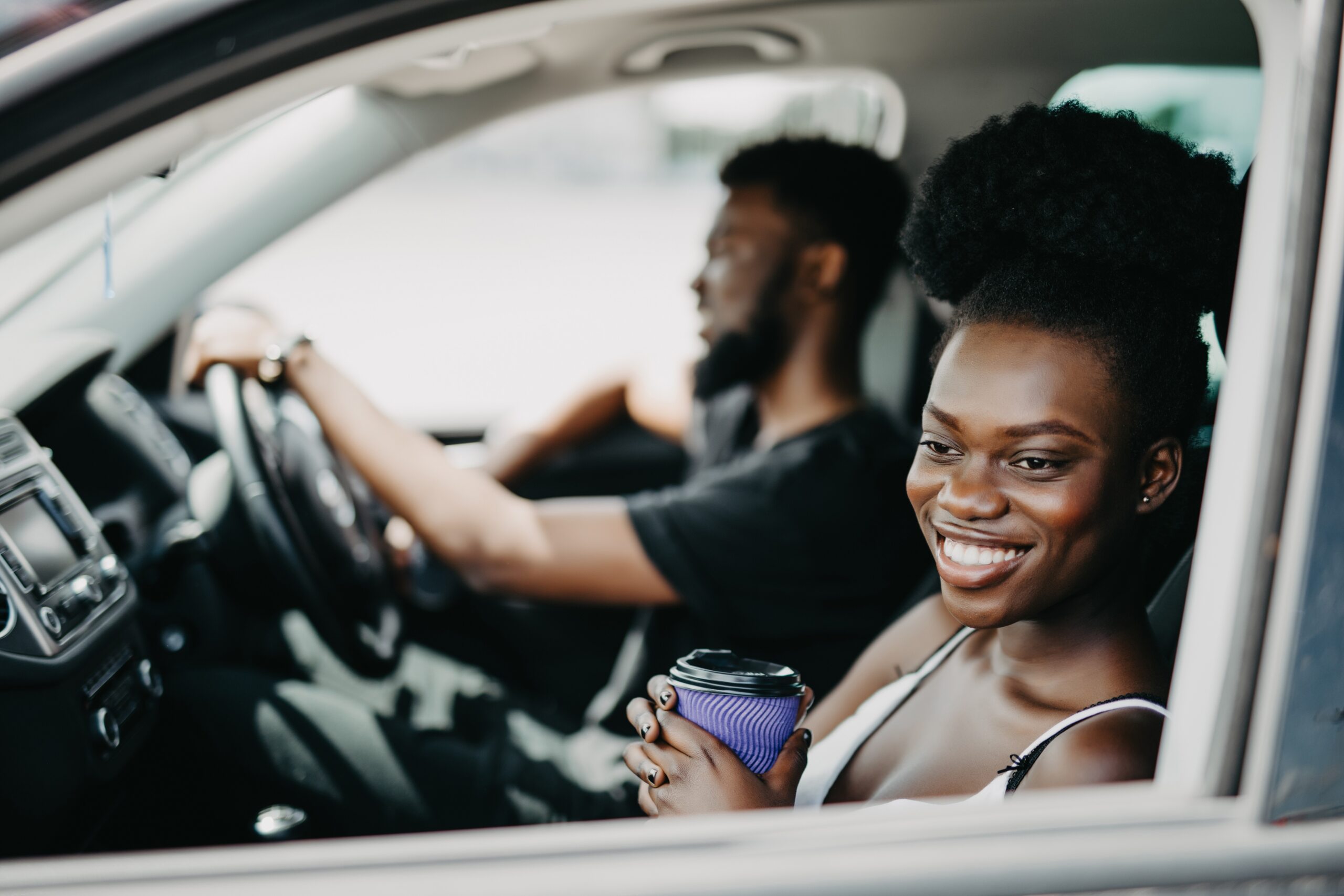
Thoughtful Driving
Earn up to 3,000 Nectar points when you share a lift and authenticate your journey in the Mobilityways app, as part of the Liftshare x Esso Thoughtful Driving Campaign.
You'll earn 1,000 Nectar points for one trip authentication, plus an additional 2,000 Nectar points for 10 trip authentications made between 26th June and 2nd October 2024.
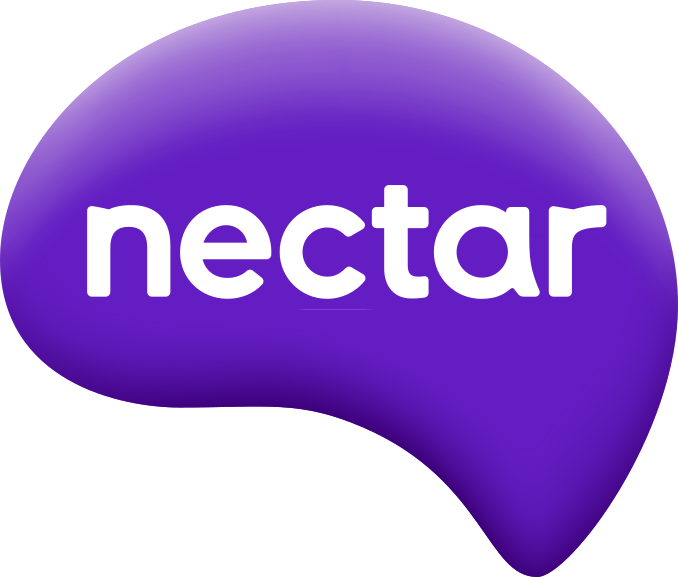
Download the Mobilityways app to get started and earn those Nectar points. Learn more about Thoughtful Driving
Find out who's travelling your way
Liftshare shows a suggested contribution for your journey based on distance and how many people are sharing. Any payment is arranged with your sharer.
Love to # liftshare
Read more liftshare member stories
Follow the Liftshare story
A to better off.
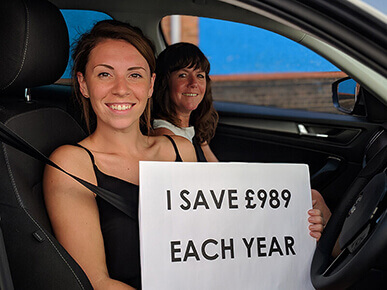
Liftsharing saves our average members over £1,000 per year
Find out why we're trusted by more than 700 corporate communities
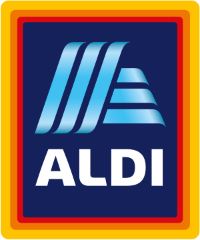
Liftshare is brought to you by Mobilityways, which is on a mission to make zero carbon commuting a reality.
Thank you for visiting nature.com. You are using a browser version with limited support for CSS. To obtain the best experience, we recommend you use a more up to date browser (or turn off compatibility mode in Internet Explorer). In the meantime, to ensure continued support, we are displaying the site without styles and JavaScript.
- View all journals
- My Account Login
- Explore content
- About the journal
- Publish with us
- Sign up for alerts
- Open access
- Published: 27 October 2022
Impacts of shared mobility on vehicle lifetimes and on the carbon footprint of electric vehicles
- Johannes Morfeldt ORCID: orcid.org/0000-0002-3618-1259 1 &
- Daniel J. A. Johansson ORCID: orcid.org/0000-0002-9138-1384 1
Nature Communications volume 13 , Article number: 6400 ( 2022 ) Cite this article
8273 Accesses
14 Citations
31 Altmetric
Metrics details
- Energy conservation
- Environmental impact
Shared cars will likely have larger annual vehicle driving distances than individually owned cars. This may accelerate passenger car retirement. Here we develop a semi-empirical lifetime-driving intensity model using statistics on Swedish vehicle retirement. This semi-empirical model is integrated with a carbon footprint model, which considers future decarbonization pathways. In this work, we show that the carbon footprint depends on the cumulative driving distance, which depends on both driving intensity and calendar aging. Higher driving intensities generally result in lower carbon footprints due to increased cumulative driving distance over the vehicle’s lifetime. Shared cars could decrease the carbon footprint by about 41% in 2050, if one shared vehicle replaces ten individually owned vehicles. However, potential empty travel by autonomous shared vehicles—the additional distance traveled to pick up passengers—may cause carbon footprints to increase. Hence, vehicle durability and empty travel should be considered when designing low-carbon car sharing systems.
Similar content being viewed by others
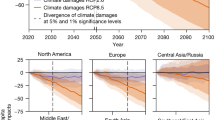
The economic commitment of climate change
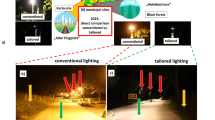
Reducing the fatal attraction of nocturnal insects using tailored and shielded road lights
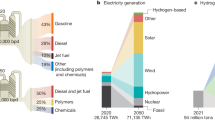
The refinery of the future
Introduction.
Decarbonizing road transportation is an important step in achieving the Paris Agreement 1 , with battery electric vehicles (BEVs) being one of the main strategies considered 2 , 3 . Transitioning towards a fully electrified passenger car fleet effectively eliminates tailpipe carbon dioxide (CO 2 ) emissions and has the potential to significantly reduce lifecycle CO 2 emissions 4 . Nevertheless, social and environmental sustainability concerns have been raised related to battery manufacturing and the mining of raw materials 5 .
Pathways with low resource exploitation and high energy efficiency are beneficial for decarbonization since they reduce the overall energy demand and material requirements. Options for passenger cars include various on-demand mobility schemes (including ride sourcing and ride sharing) that could replace individual passenger car ownership 6 , 7 , 8 . Implementing such schemes on a large scale would probably depend on self-driving (autonomous) vehicles 9 , 10 . Autonomous vehicles could decrease costs and increase the convenience of such schemes thus rendering it preferable over individually owned cars (including other arrangements where the car is primarily used by one household) 9 .
Car sharing and ride sharing could increase resource efficiency and reduce the environmental load of the system by replacing on the order of ten individually owned cars per shared car 11 . At the same time, the shared cars will likely be used more intensively during their lifetimes as compared to individually owned cars 12 . Moreover, shared autonomous vehicles may travel around without passengers for a large extent of their cumulative lifetime distance (so called “empty travel” or “deadhead travel”), which could lead to faster vehicle fleet turnover and increased manufacturing-phase emissions 13 . Simulation studies of shared autonomous vehicles have found that empty travel could increase the total vehicle travel distance by 10 to 100% in urban areas compared to the intended travel distance (i.e., the distance traveled by the car to transport a passenger or a group of passenger from one point to another) 11 , 14 . Empirical studies show a level of around 60% for taxi rides 15 and 40% for ride-sourcing services 16 , 17 on top of the intended (or served) travel distance.
The cumulative driving distance over the vehicle’s lifetime is an important assumption when estimating the carbon footprint of passenger car travel but varies significantly among studies 18 and has been shown to have large impacts on the results 19 . This assumption is even more uncertain for future mobility schemes, including systems based on car sharing or ride sharing 20 , 21 . Nevertheless, carbon footprint studies tend to assume that shared autonomous BEVs would travel at least as far as current taxis over the course of their lifetimes 12 , 13 , 22 , 23 . Hence, considering a relationship between driving intensity and vehicle lifetime is critical when assessing the carbon footprint of shared autonomous BEVs.
Studies using survival analysis 24 , 25 have determined that both calendar age and cumulative driving distance are important for the decision to retire a vehicle. Studies using statistical analyses of historical data have also shown that changes in driving intensity over the lifetime of the vehicle can have impacts on CO 2 emissions 26 and that vehicle lifetime extensions can result in lower carbon footprints 27 . However, to our knowledge, no study has yet attempted to establish a relationship between driving intensity and vehicle lifetime, and the implications of such a relationship on carbon footprints. Moreover, the carbon footprint-related consequences of changes in driving intensity in response to shared autonomous BEVs and plausible levels of empty travel have not yet been analyzed in situations where energy systems are decarbonized over time. To meet the goals of the Paris Agreement, shifts towards low-carbon manufacturing processes and electricity mixes used for charging needs to happen over the course of the next 30 years 2 , 4 . Thus, the vehicle’s lifetime, its annual driving intensity, and its interaction with decarbonizing energy systems will play important roles for the carbon footprint of passenger car travel over the coming decades.
In this work, we aim to bridge this research gap by estimating the impact of vehicle lifetime and annual driving intensity on the carbon footprints of passenger cars used for sharing. We design a semi-empirical lifetime-intensity model for assessing the lifetime of passenger cars with increasing annual driving intensity. The model is used together with prospective lifecycle assessment using vehicle fleet turnover simulations to assess the carbon footprint impacts of shared autonomous BEVs and potential levels of empty travel. The effects of climate change mitigation in global vehicle manufacturing and electricity generation are considered in the assessment. These energy and industrial systems are assumed to decarbonize in line with the Paris Agreement’s goals for the results presented in the main article, while results for an alternative pathway in line with currently stated policies are presented in the Supplementary Information. We show that the carbon footprint depends on the cumulative driving distance, which depends on both driving intensity and calendar aging. Higher driving intensities generally result in lower carbon footprints due to increased cumulative driving distance over the vehicle’s lifetime. Shared cars could decrease the carbon footprint by about 41% in 2050, if one shared vehicle replaces ten individually owned vehicles. However, potential empty travel by autonomous shared vehicles—the additional distance traveled to pick up passengers—may cause carbon footprints to increase. Hence, vehicles should be designed for durability, and empty travel should be kept low, to enhance the carbon footprint benefits of sharing.
Vehicle lifetimes decrease with increased driving intensity
Statistics on vehicle retirement can provide insights into how vehicle lifetimes vary with driving intensity. Most Swedish vehicles retired in 2014–2018 had a lifetime between 7 and 26 years and lifetime driving distances between 43 and 390 thousand kilometers (km) (95% interval). Calculating the average annual driving intensity for these vehicles results in a range between 0.5 and 28 thousand km per year (95% interval). All vehicles analyzed are internal combustion engine vehicles (ICEVs) since we are interested in capturing the behavior of mature vehicle technologies; very few BEVs have been retired so far.
The statistics show an average lifetime of 16.3 years, average lifetime driving distance of 216 thousand km, and an average annual driving intensity of 14.2 thousand km per year. Note that while a normal distribution can approximate vehicle lifetimes well, lifetime distances may be better approximated by a Weibull distribution, see Supplementary Figs. 2 – 4 , confirming previous research 14 . Since the sample is unevenly distributed over driving intensities with a bias towards the mean, stratification is used as a starting point for characterizing how the vehicle lifetimes vary with average annual driving intensity, see Fig. 1 and details on the stratified samples in Supplementary Table 4 .
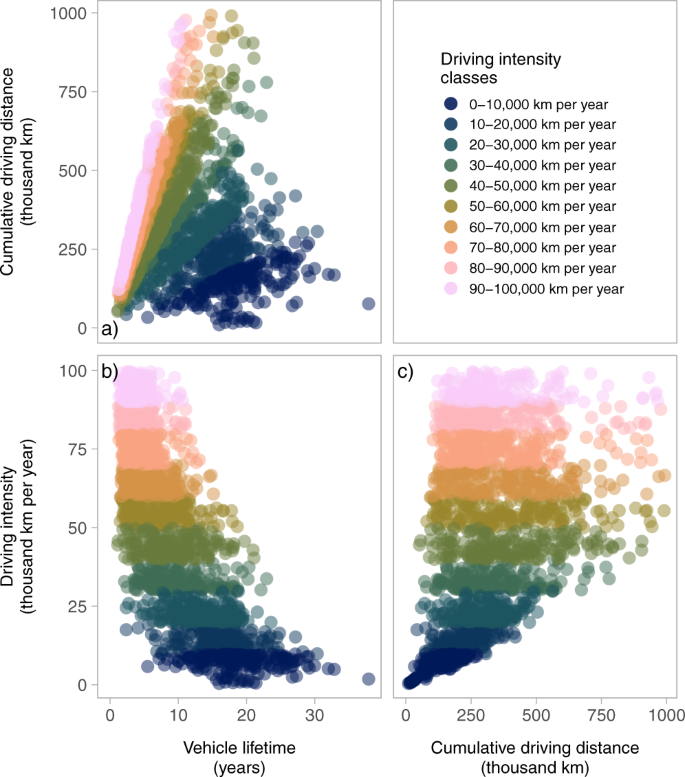
a Vehicle lifetime and cumulative driving distance. b Vehicle lifetime and average driving intensity. c Cumulative driving distance and average driving intensity. Results are shown for stratified samples based on average annual driving intensity classes of Swedish ICEVs retired between 2014 and 2018. The color indicates the driving intensity class of the data point.
The stratification is made for individual average annual driving intensity classes, varying from 0 to 100,000 km per year in steps of 10,000 km per year. For each individual driving intensity class, a close to linear relationship exists between vehicle lifetime and cumulative driving distance. The linear slope becomes steeper with each higher driving intensity class, see Fig. 1a . This suggests that the calendar age of a vehicle becomes generally shorter with increasing annual driving intensity. Further, the cumulative driving distances are distributed across a wide range for higher driving intensity classes, see Fig. 1c , while the distribution is narrower for lower driving intensities. Hence, the probability distribution of retirement becomes wider as the annual driving intensity increases, which means that the probability of a retirement decision at a specific cumulative driving distance becomes smaller. Finally, the distribution of vehicle lifetimes becomes narrower and shifts towards lower vehicle lifetimes as the average driving intensity increases, see Fig. 1b . Hence, we focus the following analysis on empirically describing the relationship between driving intensity and vehicle lifetime in order to capture the impact of vehicle use on retirement age. The data presented here does not corroborate the assumption of a fixed cumulative driving distance, which is assumed in many lifecycle assessments of vehicles 13 , 18 .
The average vehicle lifetime decreases with each higher driving intensity class, from 19 years for average driving intensities of 0–10,000 km per year to 3.9 years for average driving intensities of 90,001–100,000 km per year, see Fig. 1b . The standard deviation of the distributions also indicates that the range of probable lifetimes becomes narrower with increasing annual driving intensity (although the standard deviation increases in relative terms). The standard deviation decreases from 5.0 years for driving intensities of 0–10,000 km per year to 1.9 years for driving intensities of 90,001–100,000 km per year. Results for a categorization in four vehicle sizes (mini, medium, large, and luxury size cars, see Supplementary Fig. 5 ) suggest that cars with low annual driving intensity are mainly represented by small size cars, while large to luxury size cars mainly have higher annual driving intensities. Medium size cars cover the full spectrum of annual driving intensities.
Currently, battery degradation is often raised as a constrain to the cumulative driving distance and lifetime of BEVs 28 , 29 , 30 , but the BEV is a relatively new technology on the market and, hence, statistics on battery lifetimes from real-world driving are scarce. The number of electric vehicles on the world’s roads were in the thousands in 2010 and grew rapidly to reach about 2 million by 2016 and over 10 million by 2020 31 , 32 . Hence, if enough retirement statistics for electric vehicles were available to make thorough statistical analyses, most vehicles would be much less than 10 years old. However, the limited data currently available on cars with batteries in Swedish vehicle retirement statistics show similar distributions as the stratified data presented above, see Supplementary Notes 1 – 3 and Supplementary Figs. 11 , 12 . The data show shorter lifetimes on average (due to the limited historic data on electrified vehicles) and with a bias towards hybrid electric vehicles (HEVs) due to very few BEVs and plug-in hybrid electric vehicles (PHEVs) having been retired during the analyzed period.
Many BEV manufacturers already have warranties for their batteries of about seven to eight years or about 150,000 to 240,000 km, whichever comes first 33 , 34 , 35 , 36 , 37 . Future battery chemistries may further reduce degradation. Some studies suggest that future batteries may have significantly longer lifetimes than today. This is expected in response to altered battery chemistries 38 , changes in charging and use behavior 39 , and/or changed battery design 40 . Those changes could potentially yield a cumulative driving distance of more than three million kilometers—effectively outliving the rest of the vehicle. These improvements, if they materialize, would likely improve the cycling of the batteries. However, other factors could still limit the vehicle’s lifetime 25 , such as accidents, aging of other vehicle parts (e.g., structural elements of chassis and body), economic reasons, and consumer trends. Further, the durability of the vehicle is significantly dependent on the vehicle design, material selection, and business models 41 .
In summary, the results suggest that the annual driving intensity indeed has a strong influence on vehicle lifetimes. The relationship between driving intensity and vehicle lifetime may differ between BEVs and ICEVs, but not enough data is yet available to make such an analysis thoroughly. As a consequence, the remainder of this article explores how changes in annual driving intensity may influence the carbon footprint of passenger car travel, assuming that the relationship shown for ICEVs is applicable as a proxy for individually owned and shared autonomous BEVs. We capture the uncertainty in future vehicle lifetimes of (shared and autonomous) BEVs by highlighting extreme values for the relationship between annual driving intensity and vehicles lifetime as well as the empirically estimated relationship based on ICEV retirement data.
Impact of driving intensity on fleet-wide carbon footprints
This section presents carbon footprint estimates for BEVs at different average annual driving intensities based on the developed semi-empirical lifetime-intensity model, see Methods section for full description and discussion of the design. The model estimates the expected lifetime of a vehicle given a certain assumed average annual driving intensity. As we discussed in the previous section and in Supplementary Notes 1 – 3 , it is assumed that the lifetime-intensity model is representative for BEVs despite being calibrated on data for ICEVs. Note also that we assume that current average vehicles in terms of weight are representative for future systems 4 .
To capture the relationship between driving intensity and vehicle lifetime, we use the elasticity design of the lifetime-intensity model with Weibull distribution, see Eqs. ( 2 ), ( 3 ), ( 6 ) in Methods section, and elasticities ( ε ≈ −0.65 and β ≈ 0.51) in the simulations. The lifetime-intensity model is trained with empirical data (i.e., Swedish vehicle retirement statistics described in the previous section) using maximum likelihood estimation, see Supplementary Tables 6 , 7 . A lifetime-intensity elasticity of −0.65 implies that the scale of the lifetime, is reduced by about −0.65% if annual driving intensity is increased by 1%. The scale parameter can be seen as the characteristic lifetime and is close to the average lifetime. Consequently, the average cumulative lifetime driving distance increases by approximately 0.35% on average if annual driving intensity is increased by 1%.
Carbon footprints are also estimated for two extreme cases, ε = 0 and ε = −1, representing no influence of driving intensity on lifetime and full influence of driving intensity, respectively. The two extreme cases show the sensitivity of the model design to the assumed elasticity. The range represents possible cases if the model was trained on different retirement data. ε = 0 is a relevant extreme case if future individually owned and/or shared autonomous BEVs would age in a way where driving intensity has no importance in the decision to retire vehicles. This could be the case if the vehicle and battery degradation are only influenced by calendar age. ε = −1 represents a case where vehicle aging, including aging of the battery, is only dependent on the distance driven (e.g., if battery aging only depends on the number of charging cycles). This latter approach is used in many lifecycle assessments 13 , 18 , where fixed cumulative vehicle distances are assumed. Note though that β is based on the empirical data ( β ≈ 0.51) also for the extreme cases.
The impact of driving intensity on the carbon footprint of BEVs is estimated using a vehicle fleet turnover simulation set to meet a certain annual travel demand. Hence, fewer cars are needed to meet the travel demand if the average annual driving intensity per vehicle increases, see details in Methods. The carbon footprint is presented as emissions per vehicle-kilometer, based on the average annual emissions for a given year, including emissions from electricity used for charging and vehicle manufacturing, divided by the travel demand of that year. Figure 2 shows the results for BEVs assuming global electricity technology mix and that global manufacturing and electricity generation follow a climate change mitigation pathway in line with the goals of the Paris Agreement. The assumed pathways for carbon intensities of electricity generation used for charging are shown in Supplementary Fig. 1 .
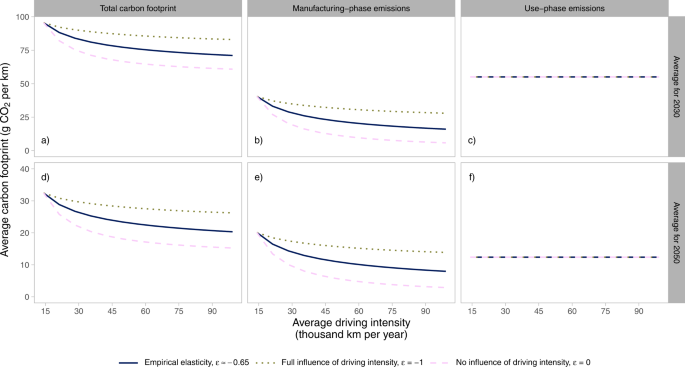
Results show the impact on annual average carbon footprints for meeting a certain travel demand: total carbon footprint ( a , d ), manufacturing-phase emissions ( b , e ), and use-phase emissions ( c , f ) for 2030 ( a – c ) and for 2050 ( d – f ), depending on the elasticity of the semi-empirical lifetime-intensity model (solid: empirical elasticity, ε ≈ −0.65, dotted: full influence of driving intensity, ε = −1, and dashed: no influence of driving intensity, ε = 0). The results assume that global manufacturing and electricity generation decarbonize in line with the Paris Agreement’s goals.
Emissions per vehicle-kilometer related to vehicle manufacturing decrease with increasing driving intensity in all cases, see Fig. 2b, e . The reason is that increased average annual driving intensity results in that fewer cars are needed to meet the travel demand. Emissions per vehicle-kilometer in the use-phase are constant for all cases since total use-phase emissions are proportionate to the travel demand, see Fig. 2c, f . Intuitively, average use-phase emissions depend on the vehicle-specific energy use and the carbon intensity of the electricity mix used for charging in each specific year, which can be seen when comparing the level in Fig. 2c, f . Hence, the total emissions per vehicle-kilometer varies with manufacturing emissions when increasing the driving intensity, see Fig. 2a, d .
As expected, manufacturing-phase emissions decrease rapidly and approach zero with increasing driving intensity when vehicle retirement is unaffected by driving intensity, i.e., ε = 0, displayed as dashed lines in Fig. 2 . This is due to the retirement decision solely depending on the calendar age when ε = 0, which is based on the empirical calendar age distribution in the model. This can be compared to when vehicle retirement is largely affected by the driving intensity, i.e., ε = −1, displayed as dotted lines in Fig. 2 . In this case, the driving distance over the whole lifetime of each vehicle is close to independent of the driving intensity. Hence, the reduction in the number of cars needed to meet the travel demand when the annual driving intensity increases would be counteracted by the number of retired vehicles that reach their maximum cumulative driving distance. This results in an inflow of new vehicles needed to replace the retired ones, which is close to independent of the driving intensity. The reasons that the number of vehicles slightly drop with increasing intensity when ε = −1 are the characteristics of the Weibull distribution, how it shifts as the intensity increases, and that the annual driving intensity for each individual car is assumed to drop by 4.4% per year. The significance of the drop in the annual driving intensity is tested in Supplementary Fig. 9 , showing that manufacturing emissions decrease less when the driving intensity is assumed to be constant over the lifetime of each vehicle.
A lifetime-intensity elasticity based on empirical evidence, i.e., ε = −0.65, results in a development in-between the two extremes, displayed as solid lines in Fig. 2 . A sensitivity analysis shows that the shape of the curves for total carbon footprint are scaled but similar in relative terms when assuming average Swedish or European Union (EU) electricity for charging, see Supplementary Fig. 9 . Further, the general pattern of the relationship between average annual driving intensity and the carbon footprint is similar also if energy systems and global manufacturing do not decarbonize in line with the Paris Agreement and instead develops according to stated policies, see Supplementary Fig. 9 .
To summarize, our results show that measures intended to increase annual driving intensity of individual cars to meet a given travel demand would result in carbon footprint reductions. Such measures include car sharing services, e.g., existing ride sourcing systems and future systems using shared autonomous BEVs. Such services could replace individual car ownership, but they may also increase driving distances because of empty travel to pick up passengers. This risk is evident for current taxis and ride sourcing services 15 , 16 , 17 as well as in simulations of future transport systems using shared autonomous vehicles 11 , 14 . In the next section, we explore how empty travel could impact the carbon footprint when simultaneously considering the possible influence that increased driving intensity might have on the lifetime of vehicles.
Empty travel by autonomous vehicles may increase emissions
The risk of empty travel when using on-demand mobility services, including those provided by autonomous vehicles, could reduce the resource and environmental efficiency of sharing. The lifetime-intensity model shows that the lifetime of the vehicle is likely to decrease with increased annual driving intensity. Vehicles may need to be replaced more often if a large part of that annual driving intensity is made up by empty travel, with increasing emissions in vehicle and battery manufacturing as a result 13 . Here, we explore how the carbon footprints of individually owned BEVs, individually owned autonomous BEVs and shared autonomous BEVs depend on the elasticity of the lifetime-intensity model and the share of empty travel. Further, we estimate the breakeven level of empty travel, i.e., the point where the carbon footprints of a fleet of shared autonomous BEVs and one of individually owned BEVs (without any empty travel) are equal.
The impact of empty travel on the carbon footprint for a fleet of shared autonomous BEVs is analyzed using the vehicle fleet turnover simulation, see details in Methods section. Simulations are made for assumptions on additional empty travel on top of the intended travel—ranging from 0 to 100%, and for assumptions on how many individually owned BEVs a shared autonomous BEV replaces—five or ten, while still meeting the given level of annual travel demand. Specific energy use per km is assumed to be the same irrespective of if the car is autonomous or not. Note though that the combination of a shared autonomous BEV replacing ten individually owned BEVs and assuming 100% empty travel results in high annual driving intensity of ca 280,000 km, which may not be possible to achieve for one car. Hence, such extreme combinations are included only for illustrative purposes. We also analyze one case with individually owned autonomous BEVs that are not shared but still may travel empty. This can occur, for example, when autonomously parking and/or charging at remote spots.
In the case where an individually owned autonomous BEV causes empty travel, the breakeven level occurs at 0% as expected, see Fig. 3a, d . This means that any empty travel caused by using the autonomous BEV results in an increase in the average carbon footprint, as compared to using a regular BEV to meet the same travel demand. In the case where individually owned BEVs are replaced by shared autonomous BEVs, we first note that a system with shared autonomous BEVs in 2030 reduces the carbon footprint per intended km traveled if no empty travel is assumed. The carbon footprint decreases from 96 g CO 2 per km for individually owned BEVs to 74 and 69 g CO 2 per km if one shared autonomous BEV replaces five or ten individually owned BEVs, respectively, assuming empirical elasticity for the lifetime-intensity model. The corresponding numbers for 2050 are 32 g CO 2 per km for individually owned BEVs, and 22 and 19 g CO 2 per km if one shared autonomous BEV replaces five or ten individually owned BEVs, respectively.
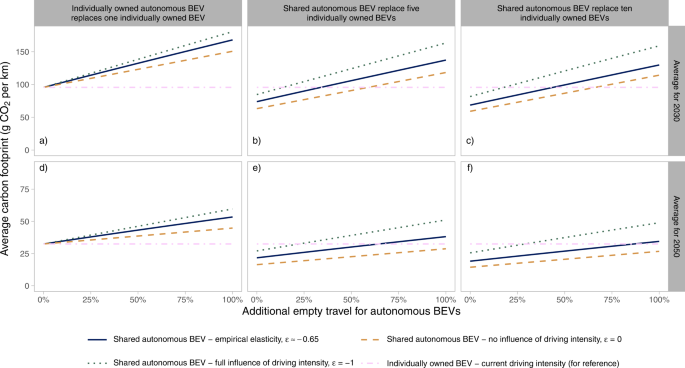
Estimated based on the average carbon footprint of individually owned autonomous BEVs that replace one individually owned BEV ( a , d ) and shared autonomous BEVs replacing five ( b , e ) or ten ( c , f ) individually owned BEVs depending on the elasticity of the semi-empirical lifetime-intensity model (solid: shared autonomous BEV – empirical elasticity, ε ≈ −0.65, dotted: shared autonomous BEV – full influence of driving intensity, ε = −1, dashed: shared autonomous BEV – no influence of driving intensity, ε = 0, and dot-dashed: individually owned BEV – current driving intensity). a – c Show results for 2030 and d – f show results for 2050. The results assume that global manufacturing and electricity generation decarbonize in line with the Paris Agreement’s goals.
The breakeven level of empty travel for a fleet in 2030 occurs at 34 and 44% for shared autonomous BEVs that replace five and ten individually owned BEVs, respectively, see intersection between solid and dot-dashed lines in Fig. 3b, c . As global manufacturing and electricity generation decarbonize further, additional levels of empty travel are possible before breakeven with the carbon footprint of individually owned BEVs is reached. Hence, for a fleet in 2050, the breakeven level of empty travel increases to 64% and 87% for shared autonomous BEVs that replace five and ten individually owned BEVs, respectively, Fig. 3e, f .
As discussed in the previous section, only manufacturing-phase emissions are affected by the lifetime-intensity model. A larger negative elasticity implies a larger inflow and outflow of shared autonomous BEVs in each year, implying a larger average carbon footprint, see Supplementary Fig. 8 . The elasticity representing no influence of driving intensity on vehicle lifetime ( ε = 0) results in higher breakeven levels as compared to the elasticity based on empirical evidence. In this case, for 2030, the breakeven level is 59 and 66% for shared autonomous BEVs that replace five and ten BEVs, respectively, see dashed lines in Fig. 3b, c , and over 100% in 2050, see dashed lines in Fig. 3e, f . Conversely, the elasticity representing full influence of driving intensity on vehicle lifetime ( ε = −1) results in lower breakeven levels. In this case for 2030, 14 and 18% for shared autonomous BEVs that replace five and ten individually owned BEVs, respectively, see dotted lines in Fig. 3b, c , and 22 and 29% for 2050, respectively, see dotted lines in Fig. 3e, f .
A sensitivity analysis shows lower breakeven levels if global manufacturing and electricity generation follows a pathway in line with stated policies rather than one that achieves the goals of the Paris Agreement, see Supplementary Fig. 10 . It also shows that the breakeven level is significantly higher, above 100% in several cases, if lower carbon intensities are assumed for electricity used for charging (i.e., Swedish or European average electricity). The sensitivity of the assumed driving intensity decrease rate of 4.4% is also tested, showing higher breakeven levels for the additional empty travel with higher driving intensity decrease rates (i.e., when a larger share of the travel for one vehicle is concentrated to early years in the vehicle’s lifetime), while the opposite holds if the driving intensity is constant over time. Nevertheless, the assumed elasticity in the lifetime-intensity model has a higher impact on the results than the assumed driving intensity decrease rate.
The significance of the elasticity in these results points to the importance of designing future shared autonomous BEVs for durability. The reason for this can be summarized: the smaller the reduction in lifetime when increasing driving intensity, the larger the potential carbon footprint benefits of car sharing.
The passenger transport systems are likely to go through several changes during the coming decades. The most prominent changes include increased use of electrified and autonomous vehicles as well as on-demand mobility schemes, including car sharing and ride sharing. These trends will affect the pathways towards decarbonization of passenger car travel, including changes in charging patterns 13 , cost structures 9 , and the value of travel time 42 , 43 , 44 , which may induce additional travel activity 45 and cause modal shifts 46 , 47 . These trends may also cause changes in vehicle design, including materials used in manufacturing 48 and changes to facilitate material recycling 49 , but many of these aspects are yet to materialize.
Our analysis shows that the relationship between vehicle lifetime and driving intensity is an important factor when estimating the carbon footprint of shared mobility. Some analysts argue that passenger cars in today’s fleets are not being used enough to compensate for material use and emissions during the manufacturing phase 49 , 50 . Therefore, increasing the driving intensity, for example through shared autonomous BEVs, may be an option for reducing lifecycle emissions from passenger car travel. However, if increasing driving intensity also results in shortened vehicle lifetimes, as suggested by the statistics, the carbon footprint would not drop as much as if a fixed lifetime were assumed.
The statistical analysis and the results from the designed semi-empirical lifetime-intensity model suggest that increased intensity of vehicle use tends to increase the cumulative lifetime distance. Hence, the results indicate that shared autonomous BEVs would reduce the carbon footprint if it results in higher driving intensity of each individual vehicle. For example, we find that a system with shared autonomous BEVs can decrease the carbon footprint per kilometer of intended travel by about 41% if one shared vehicle replaces 10 individually owned vehicles in 2050. However, this assumes a level of zero empty travel. We show that the potential carbon footprint benefit can be reduced—and even erased—if the level of empty travel becomes large. Further, besides avoiding excessive empty travel, the emissions reduction potential of shared mobility could be further improved if ride sharing is adopted, since each traveler sharing the ride in that case would bear part of the carbon footprint by effectively increasing the occupancy ratio. Note that induced travel by autonomous BEVs (both individually owned and shared) has not been assessed in this study. Nevertheless, this risk is important to consider since the use of autonomous vehicles may substantially increase the travel demand. Autonomous vehicles may effectively reduce the value of travel time and the generalized travel cost 45 since the driver does not need to be attentive and can instead use their time in the vehicle for whatever they find convenient. The potential increase in the travel demand that may follow from reduced value of travel time could further increase the total carbon footprint for the fleet as a whole.
Finally, our conclusions rely on the assumption that the relationship between driving intensity and vehicle lifetime established in the semi-empirical model will hold also for future regular and autonomous BEVs. In this article, we present preliminary evidence suggesting that cars with batteries follow similar trends as ICEVs, but the design and lifetimes of future batteries and vehicles are highly uncertain. Hence, the intention with the analysis presented in this paper is to highlight potential consequences based on currently available data and discuss them in relation to alternative assumptions. Those alternative assumptions highlight a range of plausible outcomes if the lifetime characteristics of future batteries and vehicles may deviate from those of current passenger cars. Nevertheless, the analysis shows that the carbon footprint may be substantially reduced if the relationship between average annual driving intensity and vehicle lifetime is weakened, pointing to the importance of designing future BEVs (both autonomous and regular) for durability.
Swedish vehicle retirement statistics
Statistics on Swedish passenger cars retired between 2014 and 2018 are used to understand how changes in annual average driving intensity could influence vehicle lifetimes. The statistics are collected from the Swedish registry for road transport vehicles, regulated by Swedish law 51 . The excerpt, provided by the Swedish government agency Transport Analysis 52 , includes information on the manufacturing year, date of registration, car manufacturer, engine type, mass in running order, cumulative distance traveled at last inspection, date of last inspection, and date of deregistration. The excerpt only includes vehicles that were indeed retired at the date of deregistration. Hence, vehicles that were deregistered for administrative reasons or exported are excluded.
The filtered dataset includes 442,395 observations. The filtering performed by the authors aims to reduce bias in the results and applies the following criteria: (i) age or distance traveled must not be missing, equal to zero, or equal to 999,999, (ii) time between last inspection and date of deregistration must not be longer than 14 months, (iii) time between first registration of the vehicle and the manufacturing year must not be longer than two years, (iv) average distance traveled must not be greater than 600 km per day, (v) average distance traveled must not be less than 1 km per day, (vi) mass in running order must not be greater than 3000 kg, and (vii) engine type is gasoline or diesel without hybridization, ethanol or natural gas/biogas. Details and rationale for these criteria are provided in Supplementary Tables 1 – 3 . Criterion (ii) filters many observations but including them does not significantly impact the results.
Stratified random sampling is used to create a new dataset for analyzing the influence of increasing driving intensity since only a small share of the dataset represents cars with high average annual driving intensity, such as taxis or other commercial vehicles. The strata and random sample size are set to maximize the amount of information about vehicles with high driving intensity while also ensuring high enough sample size to enable further statistical analysis. This results in strata for average annual driving intensity classes of 10,000 km/year increments from 0 km/year to 100,000 km/year. The random sample size in each stratum is 200 observations, except for the highest intensity class where the whole sample of 145 observations is used, see Supplementary Table 4 .
Semi-empirical lifetime-intensity model
The semi-empirical lifetime-intensity model enables estimations of vehicle lifetime probabilities for a given annual average driving intensity. The model can easily be updated with new parameters on average vehicle retirement lifetime, its standard deviation, and the average annual driving distance, as new statistics become available. The model can also easily be recalibrated based on new stratified random sampling datasets to enable use for other geographical regions. Two model designs are considered together with two assumptions on the probability distribution of the lifetime data as a result of these prerequisites.
If the data is assumed to follow a normal distribution, we assume that the probability of a vehicle manufactured at year t 0 , with average annual driving intensity D , being retired at year t is
In the elasticity design, we introduce a factor dependent on the quota between the annual driving intensity of the vehicle and the average annual driving intensity of current vehicle retirements, D 0 , as part of the mean,
that adjusts the expected vehicle lifetime of current retirements, \({{{\tau }}}_{0}\) , dependent on the elasticity, \({{\varepsilon }}\) , that decides the level of influence of the driving intensity. An elasticity of −1 implies that the vehicle lifetime is fully determined by the driving intensity (e.g., if driving intensity is doubled, lifetime is halved), 0 indicates no influence and the lifetime is only determined by calendar age, while an elasticity above 0 would imply that the vehicle lifetime increases with driving intensity. This design benefits from easy interpretation, but it only applies for driving intensities equal to or greater than the current average, see Fig. 4 .
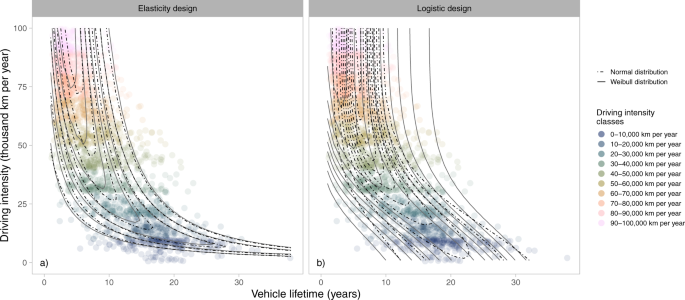
a Results for the elasticity design. b Results for the logistic design. The contours show probability density levels and are provided for both Normal (dot-dashed) and Weibull distributions (solid). Stratified samples of Swedish vehicle retirement statistics for 2014–2018 are provided in the background for comparison. The color indicates the driving intensity class of the data point.
The standard deviation,
is designed in a similar way to the design for the mean, where the constant \(\alpha=\frac{{\sigma }_{0}}{{\tau }_{0}}\) is determined based on a fit of a normal distribution to current vehicle retirement statistics. An additional elasticity, \({{\beta }}\) , is introduced in the standard deviation to account for the distributions becoming increasingly narrow with higher driving intensity classes, see Fig. 1b .
In the logistic design, we instead assume that the distribution is governed by a function inspired by the logistic curve to better capture the form of the stratified random sampling. The logistic curve function is slightly altered to reduce the number of parameters to fit to the data. Hence, \({{\mu }}\left({{D}}\right)\) and \({{{{{\rm{\sigma }}}}}}\left({{D}}\right)\) are defined as follows in this design.
where L and L 0 are the parameters that would be calibrated based on the stratified random sampling. This design applies for all driving intensities greater than zero.
If the data are assumed to follow a Weibull distribution, we assume that the probability of a vehicle manufactured in year t 0 , with average annual driving intensity D , being retired at year t , is
where the scale, \({{{{{\rm{\lambda }}}}}}\left({{D}}\right)\) , is defined in the same way as the mean, \({{\mu }}\left({{D}}\right)\) , see Eqs. (2, 4) above, and shape, \({{k}}\left({{D}}\right)\) , is defined in the same way as the standard deviation, \({{\sigma }}\left({{D}}\right)\) , see Eqs. ( 3 , 5 ) above. Note that \({{{\tau }}}_{0}\) in this case represents the scale of current vehicle retirement statistics and that \({{\alpha }}=\frac{{{{k}}}_{0}}{{{{\tau }}}_{0}}\) is determined by fitting a Weibull distribution. The fact that the median is lower than the mean for higher driving intensity classes indicates that the distribution is more positively skewed for higher driving intensity classes. This suggests that a Weibull distribution with a longer tail towards higher vehicle lifetimes would be a better fit, confirming previous research 24 , 53 .
The parameters for the different model designs are estimated using maximum likelihood estimation, see Supplementary Table 7 . A comparison of modeled vehicle lifetimes with the stratified random samples for different driving intensity classes is presented in Fig. 4 and Supplementary Fig. 7 . The contour lines in Fig. 4 , also known as isodensity lines 54 , show how the points of equal probability density for a given vehicle lifetime shift depending on the assumed driving intensity ( y -axis) and on the model design (panel and line type). The highest probability density level is shown around the mean of the distribution, and the distance indicates the rate of change. This implies that a larger distance between the lines indicates a more spread-out distribution, analogously to on a topographic map.
Figure 4a clearly shows that the elasticity design deviates from the statistics at the average current driving intensity of 14,200 km per year and approaches an infinite lifetime as driving intensities decrease. The proposed correction for this issue is to use the logistic design, as demonstrated in Fig. 4b . However, a limitation of the logistic design is that the distribution of vehicle lifetimes is assumed to be kept constant for driving intensities higher than the stratum with highest driving intensity (i.e., higher than 100,000 km per year in this study), see Supplementary Fig. 6 . The elasticity design instead results in vehicle lifetimes that approach zero for very high driving intensities.
Regarding the choice of distribution, the Weibull distribution benefits from better reflecting the skewness of the statistics. However, it overcompensates for higher driving intensities when applied with the logistic design, resulting in longer tails of vehicle lifetimes than the statistics indicate, see the greater distance between lines in Fig. 4b . This difference between Normal- and Weibull-based model designs is close to negligible for the elasticity design. Benefits and drawbacks for the choice of distribution and model design are summarized in Table 1 .
Prospective lifecycle assessment with vehicle fleet turnover
A vehicle fleet turnover simulation is designed to evaluate the impact of lifetime-intensity elasticities on the carbon footprint for individually owned BEVs, individually owned autonomous BEVs and shared autonomous BEVs. The simulations also test assumptions on how many individual owned BEVs that a shared autonomous BEV can replace, and different levels of implied empty travel of shared autonomous BEVs.
Average carbon footprints (reported in g CO 2 per vehicle-kilometer of intended travel) are estimated for the fleet using a prospective lifecycle assessment framework based on GREET® 2 - Version 2019 55 adapted for scenario analysis 4 . The framework enables estimations of future carbon footprints of passenger cars depending on climate change mitigation efforts in electricity generation and global manufacturing. Two pathways for this mitigation are analyzed: one in line with stated policies and one that achieves the goals of the Paris Agreement. The results presented in the main paper assumes a pathway that achieves the goals of the Paris Agreement, while the results for stated policies are presented in the Supplementary Information. The stated policies pathway is based on currently implemented and stated climate policies by 2019 and the pathway in line with the goals of the Paris Agreement is designed to limit global mean temperature increase to below 1.8 °C. The two pathways are based on the IEA 56 scenarios named Stated Policies and Sustainable Development.
The vehicle fleet turnover simulation is designed for the fleet to match a constant annual travel demand equal to N 0 = 1000 cars driving at average driving intensity, D 0 = 14,200 km per year. For each year, t , the number of new cars needed are estimated by solving Eqs. ( 7 ), ( 8 ):
where the number of new cars, N , is estimated as the difference between the annual travel demand and the annual travel range of the current fleet, \(\hat{S}\) , divided by the annual vehicle-kilometers, m , for a new car.
The travel range of the current fleet, \(\hat{S}\) , in each year, t , is given by
where the fleet of the previous year is an age distribution for age cohorts, \(\widetilde{{{t}}}\) , from age 1 to 40 years, in one-year steps. The initial age distribution for the first year is estimated by a Weibull distribution (shape 1.4 and scale 13). The distribution is informed by statistics on the age of the Swedish vehicle car fleet 57 and serves to initiate the simulation, which is run for 50 iterations (years) to give it time to stabilize at a steady state level. The annual range covered by a car of a given age, \(\widetilde{{{t}}}\) , is given by
where the annual driving range is assumed to decrease by b = 4.4% per year over its lifetime (estimated based on statistics on driving distances in Sweden 57 ), D 0 represents the average annual driving intensity and τ 0 represents the mean vehicle lifetime. The retirements for each age cohort in year, t , are given by the cumulative probability distribution for the semi-empirical lifetime-intensity model, assuming the elasticity design and Weibull distribution, as described in Eqs. ( 2 ), ( 3 ), ( 6 ), assuming that the driving intensity, \(D\) , is equal to \({D}_{i}\cdot \left(1+\theta \right)\) , where D i is the intended travel distance and θ represents the additional share of empty travel. The probability of retirement earlier than a lifetime of one year is added to the probability of retirement at the one-year mark. This is to avoid truncating the probabilities for retirement for cars with a lifetime of less than one year, which is a risk for the extreme case of ( ε = −1).
The model returns annual sales, stock, and retirements. Carbon footprints per km, CF, associated with that steady state are estimated based on the total manufacturing- and use-phase emissions, E , for each year divided by the total intended traveling distance, \({N}_{0}\cdot {D}_{0}\) :
Manufacturing-phase CO 2 emissions, E Manufacturing , are estimated for car sales in each year based on manufacturing processes as implemented in GREET® for the Stated Policies Scenario, while new and innovative processes are phased in over time for the Sustainable Development Scenario based on a literature review 4 . Use-phase CO 2 emissions, E Use , are estimated annually based on total traveled distance (including potential empty travel), vehicle energy use, and appropriate carbon intensities described below. The specific energy use of the cars are assumed be 201 Wh per km 4 . Autonomous BEVs are assumed to have the same specific energy use per km as regular BEVs. BEVs are assumed to charge with electricity produced using average global, European, or Swedish technology mixes (results for European and Swedish technology mixes are presented in the Supplementary Information).
The carbon intensity of electricity is based on estimates of average direct emissions for future electricity mixes for each respective geographic area, see description of the data sources for the scenarios below. 2019 is used as a base year to avoid the influence of the Covid-pandemic on the carbon intensities. The carbon intensities used for electricity represent averages for each respective geographic area following the attributional nature of the chosen prospective lifecycle assessment framework 58 , 59 . Upstream emissions occurring in production of fuels and power stations are accounted for by adding a weighted factor for future electricity mixes based on estimates by Pehl et al. 60 . We assume that Pehl et al.’s estimates of upstream emissions for each electricity generation technology can be applied regardless of geographic area and that their baseline and climate policy scenarios resemble the Stated Policies and Sustainable Development scenarios used in this study. Note that emissions for the construction of water and nuclear power stations are assumed to be zero for Sweden and the European Union due to their long lifetime, the fact that they were mainly constructed several decades ago, and that few new stations are planned. Hence, we assume that the emissions from the construction of these stations are only attributed to electricity production prior to 2019. Continuing to account for these construction-related emissions in the carbon intensity of electricity after 2019 would not have any significant impact on the results.
For the global electricity mix used in manufacturing and for charging, future direct emissions and adjustments to account for transmission and distribution losses (based on the difference between estimated supply and demand) are based on estimates by the IEA 56 for the two decarbonization pathways, Stated Policies and Sustainable Development. For the European electricity mix used for charging, direct emissions and adjustments to account for transmission and distribution losses are based on scenarios by the European Commission 61 combined with the cap of the European Union emissions trading system reaching zero in 2058 62 for both decarbonization pathways. For the Swedish electricity mix used for charging, direct emissions for 2019 are calculated based on the total emissions for electricity generation divided by the end-use of electricity 63 , 64 . Direct emissions are assumed to decrease linearly to zero by 2045 for both decarbonization pathways, in line with the adopted net-zero emission target and the Swedish government’s intention 65 to reach zero emissions from electricity generation. Upstream emissions are based on estimates by Pehl et al. 60 combined with projections for the future electricity generation mix by the IEA 56 , European Commission 61 , and Swedish Energy Agency 66 .
Reporting summary
Further information on research design is available in the Nature Research Reporting Summary linked to this article.
Data availability
Data for all figures and additional data used in the analyses are available from the corresponding author upon request. Note that the detailed data on vehicle retirement are treated as confidential since data that could be traced back to individuals or companies are protection under the Swedish Public Access to Information and Secrecy Act (SFS 2009:400). Hence, requests for access to these detailed data should be made directly to the Swedish governmental agency Transport Analysis ( https://www.trafa.se/vagtrafik/fordon/ - dataset “Fordon på väg”).
Code availability
The computer code used to generate the results reported in this study are available from the corresponding author upon request.
United Nations Environment Programme. Emissions Gap Report 2020. https://www.unenvironment.org/emissions-gap-report-2020 (2020).
de Coninck, H. et al. Strengthening and implementing the global response. In Global warming of 1.5 °C. An IPCC Special Report on the Impacts of Global Warming of 1.5 °C above Pre-Industrial Levels and Related Global Greenhouse Gas Emission Pathways, in the Context of Strengthening the Global Response to the Threat of Climate Change (eds Masson-Delmotte, V. et al.) (IPCC, 2018).
MIT Energy Initiative. Insights into Future Mobility. http://energy.mit.edu/insightsintofuturemobility (2019).
Morfeldt, J., Davidsson Kurland, S. & Johansson, D. J. A. Carbon footprint impacts of banning cars with internal combustion engines. Transp. Res. Part D: Transp. Environ. 95 , 102807 (2021).
Article Google Scholar
International Energy Agency (IEA). Global EV Outlook 2019 - Scaling-up the transition to electric mobility. https://www.iea.org/reports/global-ev-outlook-2019 (2019).
Grubler, A. et al. A low energy demand scenario for meeting the 1.5 °C target and sustainable development goals without negative emission technologies. Nat. Energy 3 , 515–527 (2018).
Tikoudis, I. et al. Ridesharing services and urban transport CO2 emissions: Simulation-based evidence from 247 cities. Transp. Res. Part D: Transp. Environ. 97 , 102923 (2021).
Jaramillo, P. et al. Transport. In IPCC, 2022: Climate Change 2022: Mitigation of Climate Change. Contribution of Working Group III to the Sixth Assessment Report of the Intergovernmental Panel on Climate Change (eds Shukla, P. R. et al.) (Cambridge University Press, 2022).
Compostella, J. et al. Travel time costs in the near- (circa 2020) and long-term (2030–2035) for automated, electrified, and shared mobility in the United States. Transp. Policy 105 , 153–165 (2021).
Fulton, L. M. Three revolutions in urban passenger travel. Joule 2 , 575–578 (2018).
Pernestål, A. & Kristoffersson, I. Effects of driverless vehicles—Comparing simulations to get a broader picture. Eur. J. Transp. Infrastruct. Res. 19 , 1–23 (2019).
Google Scholar
Greenblatt, J. B. & Saxena, S. Autonomous taxis could greatly reduce greenhouse-gas emissions of US light-duty vehicles. Nat. Clim. Change 5 , 860–863 (2015).
Saleh, M., Milovanoff, A., Daniel Posen, I., MacLean, H. L. & Hatzopoulou, M. Energy and greenhouse gas implications of shared automated electric vehicles. Transportation Res. Part D: Transp. Environ. 105 , 103233 (2022).
Schäfer, A. W. & Yeh, S. A holistic analysis of passenger travel energy and greenhouse gas intensities. Nat. Sustainability 3 , 459–462 (2020).
Cramer, J. & Krueger, A. B. Disruptive change in the taxi business: The case of uber. Am. Economic Rev. 106 , 177–182 (2016).
Henao, A. Impacts of Ridesourcing—Lyft and Uber—on Transportation including VMT, Mode Replacement, Parking, and Travel Behavior (University of Colorado, 2017).
Ward, J. W., Michalek, J. J. & Samaras, C. Air pollution, greenhouse gas, and traffic externality benefits and costs of shifting private vehicle travel to ridesourcing services. Environ. Sci. Technol. 55 , 13174–13185 (2021).
CAS PubMed Google Scholar
Nordelöf, A., Messagie, M., Tillman, A. M., Ljunggren Söderman, M. & Van Mierlo, J. Environmental impacts of hybrid, plug-in hybrid, and battery electric vehicles—what can we learn from life cycle assessment? Int. J. Life Cycle Assess. 19 , 1866–1890 (2014).
Hawkins, T. R., Singh, B., Majeau‐Bettez, G. & Strømman, A. H. Comparative environmental life cycle assessment of conventional and electric vehicles. J. Ind. Ecol. 17 , 53–64 (2013).
Article CAS Google Scholar
Amatuni, L., Ottelin, J., Steubing, B. & Mogollón, J. M. Does car sharing reduce greenhouse gas emissions? Assessing the modal shift and lifetime shift rebound effects from a life cycle perspective. J. Cleaner Prod. 266 , 121869 (2020).
Vilaça, M., Santos, G., Oliveira, M. S. A., Coelho, M. C. & Correia, G. H. A. Life cycle assessment of shared and private use of automated and electric vehicles on interurban mobility. Appl. Energy 310 , 118589 (2022).
Fernando, C. et al. Life cycle environmental assessment of a transition to mobility servitization. Proc. CIRP 90 , 238–243 (2020).
Gawron, J. H., Keoleian, G. A., De Kleine, R. D., Wallington, T. J. & Kim, H. C. Deep decarbonization from electrified autonomous taxi fleets: Life cycle assessment and case study in Austin, TX. Transp. Res. Part D: Transp. Environ. 73 , 130–141 (2019).
Kolli, Z., Hivert, L. & Dupont-Kieffer, A. Car survival in a national fleet: A non-parametric approach based on French data. 12th World Conference on Transport Research , 11-15 July 2010, Lisbon, Portugal. https://hal.archives-ouvertes.fr/hal-00614977 (2010).
Chen, C. & Niemeier, D. A mass point vehicle scrappage model. Transp. Res. Part B: Methodol. 39 , 401–415 (2005).
Kaneko, M. & Kagawa, S. Driving propensity and vehicle lifetime mileage: A quantile regression approach. J. Environ. Manag. 278 , 111499 (2021).
Nakamoto, Y. & Kagawa, S. A generalized framework for analyzing car lifetime effects on stock, flow, and carbon footprint. J. Ind. Ecol. https://doi.org/10.1111/jiec.13190 (2021).
Yang, F., Xie, Y., Deng, Y. & Yuan, C. Predictive modeling of battery degradation and greenhouse gas emissions from U.S. state-level electric vehicle operation. Nat. Commun. 9 , 2429 (2018).
Article PubMed PubMed Central Google Scholar
Lander, L. et al. Cost and carbon footprint reduction of electric vehicle lithium-ion batteries through efficient thermal management. Appl. Energy 289 , 116737 (2021).
Baars, J., Domenech, T., Bleischwitz, R., Melin, H. E. & Heidrich, O. Circular economy strategies for electric vehicle batteries reduce reliance on raw materials. Nat. Sustain. 4 , 71–79 (2021).
International Energy Agency (IEA). Global EV Outlook 2020 - Entering the decade of electric drive? https://doi.org/10.1787/9789264302365-en (2020).
International Energy Agency (IEA). Global EV Outlook 2021 - Accelerating ambitions despite the pandemic. https://www.iea.org/reports/global-ev-outlook-2021 (2021).
Tesla. Vehicle Warranty. https://www.tesla.com/support/vehicle-warranty (2022).
Polestar. En garanti som speglar vårt tillvägagångssätt (A warranty that mirrors our approach). https://www.polestar.com/se/service-and-assistance/car-warranty/ (2022).
Volkswagen. High-voltage battery: Warranty and maintenance. 1–11 https://www.volkswagen.co.uk/en/electric-and-hybrid/should-you-go-electric/servicing/battery-maintenance-and-waranty.html (2022).
Kia. Warranty terms and exclusions. 1–8 https://www.kia.com/uk/owners/insurance-and-warranty/7-year-warranty/terms-and-exclusions/ (2022).
Hyundai. Warranties. 1–10 https://www.hyundai.com/eu/driving-hyundai/owning-a-hyundai/warranty.html (2022).
Ye, L. & Li, X. A dynamic stability design strategy for lithium metal solid state batteries. Nature 593 , 218–222 (2021).
Article CAS ADS PubMed Google Scholar
Wikner, E. & Thiringer, T. Extending battery lifetime by avoiding high SOC. Appl. Sci. (Switzerland) 8 , 1825 (2018).
Yang, X. G., Liu, T. & Wang, C. Y. Thermally modulated lithium iron phosphate batteries for mass-market electric vehicles. Nat. Energy 6 , 176–185 (2021).
Bakker, C. A., Mugge, R., Boks, C. & Oguchi, M. Understanding and managing product lifetimes in support of a circular economy. J. Clean. Prod. 279 , 123764 (2021).
Rebalski, E. Are We There Yet? Combining Qualitative and Quantitative Methods to Study the Introduction of CAVs in Sweden, and Potential Travel Demand Effects (Chalmers University of Technology, 2021).
Steck, F., Kolarova, V., Bahamonde-Birke, F., Trommer, S. & Lenz, B. How autonomous driving may affect the value of travel time savings for commuting. Transp. Res. Rec. 2672 , 11–20 (2018).
de Almeida Correia, G. H., Looff, E., van Cranenburgh, S., Snelder, M. & van Arem, B. On the impact of vehicle automation on the value of travel time while performing work and leisure activities in a car: Theoretical insights and results from a stated preference survey. Transp. Res. Part A: Policy Pract. 119 , 359–382 (2019).
Wadud, Z., MacKenzie, D. & Leiby, P. Help or hindrance? The travel, energy and carbon impacts of highly automated vehicles. Transp. Res. Part A: Policy Pract. 86 , 1–18 (2016).
Meyer, J., Becker, H., Bösch, P. M. & Axhausen, K. W. Autonomous vehicles: The next jump in accessibilities? Res. Transp. Econ. 62 , 80–91 (2017).
Wadud, Z. & Chintakayala, P. K. To own or not to own—That is the question: The value of owning a (fully automated) vehicle. Transp. Res. Part C: Emerg. Technol. 123 , 102978 (2021).
Taiebat, M., Brown, A. L., Safford, H. R., Qu, S. & Xu, M. A review on energy, environmental, and sustainability implications of connected and automated vehicles. Environ. Sci. Technol. 52 , 11449–11465 (2018).
Richter, J. L. A circular economy approach is needed for electric vehicles. Nat. Electron. 5 , 5–7 (2022).
Hertwich, E. G. et al. Material efficiency strategies to reducing greenhouse gas emissions associated with buildings, vehicles, and electronics—a review. Environ. Res. Lett. 14 , 043004 (2019).
Swedish Parliament. Lag (2001:558) om vägtrafikregister (Law on road traffic registry). https://www.riksdagen.se/sv/dokument-lagar/dokument/svensk-forfattningssamling/lag-2001558-om-vagtrafikregister_sfs-2001-558 (2001).
Transport Analysis. Detailed excerpt on scrappage of vehicles from the statistics ‘Fordon på väg’ (Road vehicles) for the years 2014–2018. https://www.trafa.se/vagtrafik/fordon/ (2020).
Kızılersü, A., Kreer, M. & Thomas, A. W. The Weibull distribution. Significance 15 , 10–11 (2018).
Fernández, A. & Vázquez, M. A generalized regression methodology for bivariate heteroscedastic data. Commun. Stat. - Theory Methods 40 , 598–621 (2011).
Article MathSciNet MATH Google Scholar
Argonne National Laboratory. The Greenhouse Gases, Regulated Emissions, and Energy Use in Transportation (GREET®) Model - GREET 2, Version 2019. https://greet.es.anl.gov/ (2020).
IEA. World Energy Outlook 2020. www.iea.org/weo (2020).
Transport Analysis. Körsträckor med svenskregistrerade fordon (Mileage for vehicles registered in Sweden). https://www.trafa.se/vagtrafik/korstrackor/ (2020).
Yang, Y. Two sides of the same coin: Consequential life cycle assessment based on the attributional framework. J. Clean. Prod. 127 , 274–281 (2016).
Arvidsson, R. et al. Environmental assessment of emerging technologies: Recommendations for prospective LCA. J. Ind. Ecol. 22 , 1286–1294 (2018).
Pehl, M. et al. Understanding future emissions from low-carbon power systems by integration of life-cycle assessment and integrated energy modelling. Nat. Energy 2 , 939–945 (2017).
European Commission. In-depth analysis in support of the Commission communication COM(2018) 773 - A Clean Planet for all - A European long-term strategic vision for a prosperous, modern, competitive and climate neutral economy. https://ec.europa.eu/clima/policies/strategies/2050_en (2018).
Zetterberg, L. & Elkerbout, M. Policy Brief: The Future of the EU Emissions Trading System - Responding to the EU Green Deal Proposals. https://www.mistracarbonexit.com/s/Policy-Brief_MCE_dec2019_3.pdf (2019).
Swedish Energy Agency. Energiläget i siffror 2021 (Energy in Sweden in numbers 2021). https://www.energimyndigheten.se/statistik/energilaget/ (2021).
Swedish Environmental Protection Agency. Fördjupad analys av den svenska klimatomställningen 2020 - Klimat och luft i fokus (In-depth analysis of the Swedish climate transition 2019 - Climate and air pollution in focus). Rapport Vol. 6945 http://www.naturvardsverket.se/978-91-620-6945-2 (2020).
Swedish Government. Regeringens proposition 2019/20:65 En samlad politik för klimatet – klimatpolitisk handlingsplan (Governmental bill 2019/20:65 Joint policy for climate change - climate policy action plan). https://www.regeringen.se/rattsliga-dokument/proposition/2019/12/prop.-20192065/ (2020).
Swedish Energy Agency. Scenarier över Sveriges energisystem 2020 (Scenarios for Sweden’s energy system 2020) ER 2021:6. https://energimyndigheten.a-w2m.se/Home.mvc?ResourceId=185971 (2021).
Download references
Acknowledgements
We acknowledge the support for this research by Mistra Carbon Exit financed by Mistra, the Swedish Foundation for Strategic Environmental Research (J.M. and D.J.A.J.), and by the NAVIGATE project financed by the European Commission, H2020/2019-2023, grant agreement number 821124 (D.J.A.J.).
Open access funding provided by Chalmers University of Technology.
Author information
Authors and affiliations.
Physical Resource Theory, Department of Space, Earth and Environment, Chalmers University of Technology, Maskingränd 2, SE-412 96, Gothenburg, Sweden
Johannes Morfeldt & Daniel J. A. Johansson
You can also search for this author in PubMed Google Scholar
Contributions
J.M. and D.J.A.J. contributed jointly to conceptualizing the research and writing the article. J.M. performed the statistical analysis and computational studies.
Corresponding author
Correspondence to Johannes Morfeldt .
Ethics declarations
Competing interests.
The authors declare no competing interests.
Peer review
Peer review information.
Nature Communications thanks Han Hao and the other, anonymous, reviewer(s) for their contribution to the peer review of this work. Peer reviewer reports are available.
Additional information
Publisher’s note Springer Nature remains neutral with regard to jurisdictional claims in published maps and institutional affiliations.
Supplementary information
Supplementary information, peer review file, reporting summary, rights and permissions.
Open Access This article is licensed under a Creative Commons Attribution 4.0 International License, which permits use, sharing, adaptation, distribution and reproduction in any medium or format, as long as you give appropriate credit to the original author(s) and the source, provide a link to the Creative Commons license, and indicate if changes were made. The images or other third party material in this article are included in the article’s Creative Commons license, unless indicated otherwise in a credit line to the material. If material is not included in the article’s Creative Commons license and your intended use is not permitted by statutory regulation or exceeds the permitted use, you will need to obtain permission directly from the copyright holder. To view a copy of this license, visit http://creativecommons.org/licenses/by/4.0/ .
Reprints and permissions
About this article
Cite this article.
Morfeldt, J., Johansson, D.J.A. Impacts of shared mobility on vehicle lifetimes and on the carbon footprint of electric vehicles. Nat Commun 13 , 6400 (2022). https://doi.org/10.1038/s41467-022-33666-2
Download citation
Received : 15 February 2022
Accepted : 28 September 2022
Published : 27 October 2022
DOI : https://doi.org/10.1038/s41467-022-33666-2
Share this article
Anyone you share the following link with will be able to read this content:
Sorry, a shareable link is not currently available for this article.
Provided by the Springer Nature SharedIt content-sharing initiative
This article is cited by
Predicting building energy consumption in urban neighborhoods using machine learning algorithms.
- Qingrui Jiang
- Chenyu Huang
Frontiers of Urban and Rural Planning (2024)
Hidden delays of climate mitigation benefits in the race for electric vehicle deployment
- Xinzhu Zheng
Nature Communications (2023)
Decarbonization potential of electrifying 50% of U.S. light-duty vehicle sales by 2030
- Maxwell Woody
- Gregory A. Keoleian
- Parth Vaishnav
By submitting a comment you agree to abide by our Terms and Community Guidelines . If you find something abusive or that does not comply with our terms or guidelines please flag it as inappropriate.
Quick links
- Explore articles by subject
- Guide to authors
- Editorial policies
Sign up for the Nature Briefing: Anthropocene newsletter — what matters in anthropocene research, free to your inbox weekly.

Car Sharing
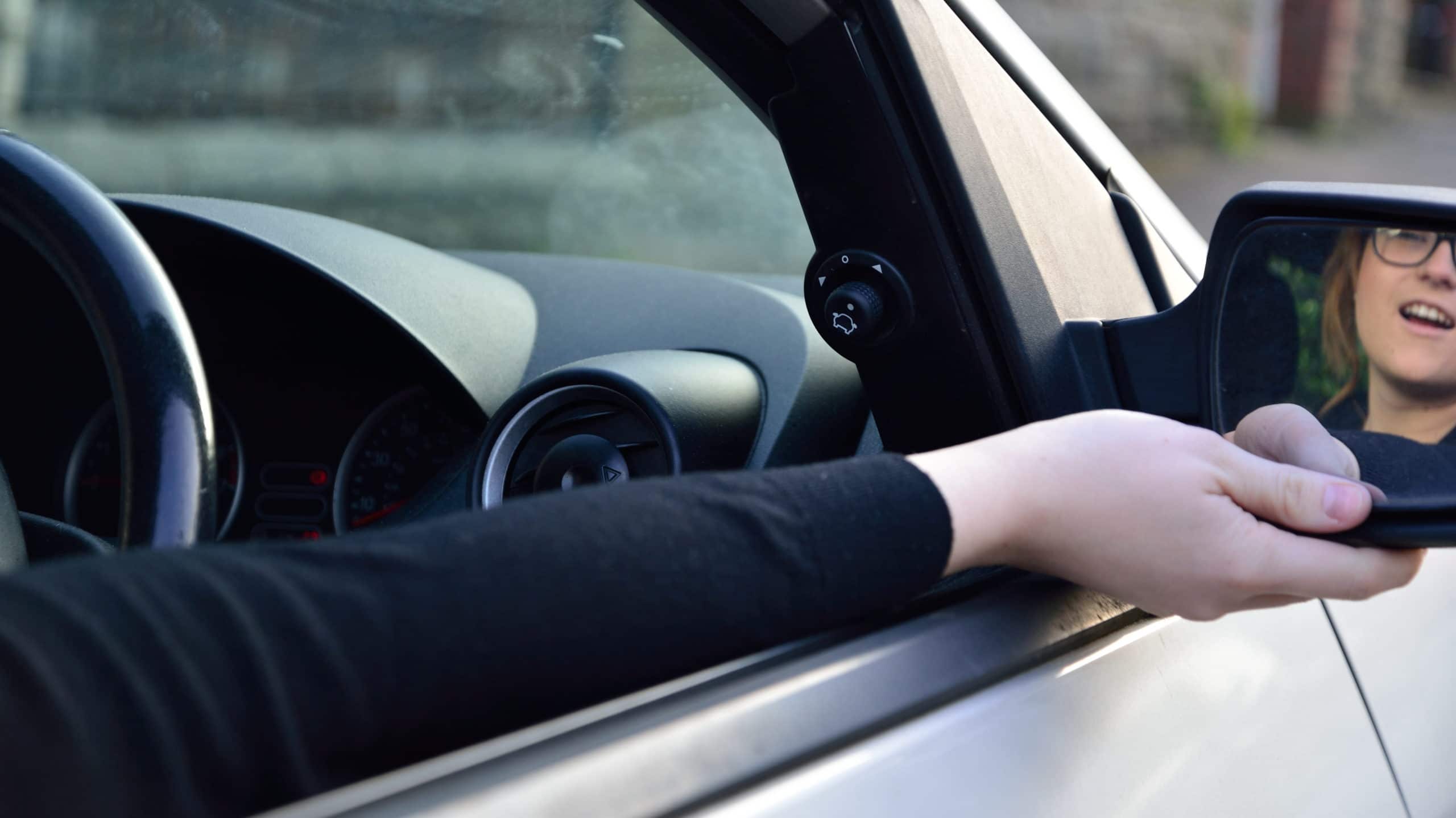
The sustainable way to travel by car, saving you money and time.
Join my journey launches for west of england commuters.
Join My Journey is a free online service that matches commuters up from the same workplace to share walking, cycling and car journeys.
Business leaders across Bristol and the West of England are now being encouraged to sign up their companies and organisations to the online platform so their staff can easily share journeys to work.
For anyone who does not work in a large company, there is also the option to join an area hub, where smaller businesses are linked up with others to share journeys to the same area.
Visit joinmyjourney.org.uk to register your organisation or join as an individual .
What is car sharing?
Car sharing is all about filling empty seats, sharing costs, and it’s a great way to meet new people. You can get to know your colleagues or new travelers going the same way.
Here are a few examples of when car sharing can be used:
- Commuting to work
- Doing the school run
- Going to college / university
- Travelling to a festival , a big event or a show
- Going to a sporting match
Is car sharing for you?
Car sharing is so easy that we can safely say that it could be a great option for everyone! You don’t even need to own a car, just search for drivers going the same way.
- Share traveling costs – significant annual savings for car owners and passengers
- Meet new people – a great way to network
- Reduce traffic – less congestion and air pollution
- Get there faster – using 2+ lanes to beat the commuting queues
- Park easily – fewer cars, so more spaces
- Workplace advantages – some employers offer dedicated parking for 2+ cars
Find a car sharing buddy today!
Here is a list of some car sharing websites where you can find people going the same way:
Disclaimer: We are not affiliated with any of the websites above unless otherwise clearly stated. The list above is for information purposes only. This should not be interpreted as an official recommendation and we take no responsibility for any of the websites listed. You must use your own judgement when deciding which website to use.
Major Alerts
Major updates that may affect your travel in the west of england..
We've detected unusual activity from your computer network
To continue, please click the box below to let us know you're not a robot.
Why did this happen?
Please make sure your browser supports JavaScript and cookies and that you are not blocking them from loading. For more information you can review our Terms of Service and Cookie Policy .
For inquiries related to this message please contact our support team and provide the reference ID below.

- Moscow Tours
- Customized tours
- Moscow for kids
- Evening activities
- Moscow evening activities
- St Petersburg evening activities
- Day trips out of Moscow
- Golden Ring tours
- St Petersburg tours
- Russian tour destinations
- Package tours
- Moscow highlights
- Travel Tips
- Upcoming group tours
- Moscow events
Carsharing in Moscow

How much does it cost?
The rental price depends on the chosen operator, the car class, traffic congestion, demand for carsharing cars, and ranges from 3 to 20 rubles per minute. When you leave the car to wait for you at the parking lot, you'll need to pay around 2 rubles per minute. The advantage of car sharing is that you do not need to spend money on parking, gasoline, insurance, car wash, and maintenance.
How to use?
To rent a car, you need to register in the operator's mobile application. During the registration process, you need to attach photos of your passport and driver's license and then sign an agreement (by joining the operator's conditions in the application). The verification process can take several days. You can book an available car in the app. Once the vehicle is reserved, you have some free booking time - 20 minutes on average - to get to the place. After the waiting time expires, you'll need to pay the waiting rate, ranging from 2 to 5 rubles per minute. The car opens through the app. All the necessary documents and the ignition key are inside the vehicle. After a quick check for damage, you can start renting.
How far to go?
In most cases, you can move around the city, get to Moscow airports, and drive around the Moscow countryside. YouDrive vehicles can drive 250 km outside the Moscow Ring Road. But there are only a few locations outside of Moscow where it is allowed to complete the lease.
Where to leave your car?
The rules are a bit different in each company. DeliMobil allows you to complete the lease in a kilometer from metro stations in Moscow, in the parking lots of the shopping center, at the airports. YouDrive The completion zone in this service changes over time. From 08:00 Mon to 20:00 Fri, the car can be parked within the Third Ring Road and next to the metro Vodny Stadion, Molodezhnaya, Kuzminki, Anino, Domodedovskaya, Yuga-Zapadnaya, Novogireevo. On weekends, the zone expands to the Moscow Ring Road. For more detailed information you'll need to check the details on the car-sharing applications.
When to use?
Please note that Moscow traffic is very busy and it takes time to get used to it. We would not recommend you using car-sharing in cases when you are in a hurry. So far it is also not the best transport for tours of Moscow . However, it might be a good solution for people willing to take a day trip outside of Moscow.

We use cookies to improve your experience on our website, and to facilitate providing you with services available through our website. By continuing to use our website, you accept our use of cookies, the terms of our Privacy Policy and Terms of Service . I agree
Future Of Travel: XPENG AEROHT Teams Up With Autoliv For Flying Car Safety Tech
XPeng Inc (NYSE:XPEV) affiliate, XPENG AEROHT, and Autoliv China, a division of Autoliv, Inc. (NYSE:ALV), have signed a strategic cooperation agreement .
The agreement is aimed at pioneering safety solutions for future mobility. The parties will collaborate on various initiatives to develop safety solutions for flying cars.
Based on their shared commitment to future mobility safety, Autoliv and XPENG AEROHT will work together on various initiatives to develop safety solutions specifically for flying cars.
“XPENG AEROHT is at the forefront in this field, and we are excited to explore new opportunities for making this new type of vehicle safe. By combining their advanced technology with our expertise in safety solutions, I believe together we can play an important role in making flying cars a reality,” said Sng Yih, President of Autoliv China.
Also Read : XPeng Faces Sales Model Challenges Amid Staffing Adjustments And Leadership Shifts: Report
XPENG AEROHT, an affiliate of electric vehicle company XPeng, is involved in flying car innovation in Asia.
Deli Zhao, Founder & President of XPENG AEROHT, stated, “This strategic partnership elevates the relationship between the two parties to a new level, representing a comprehensive upgrade of XPENG AREOHT’s supply chain.”
Price Action : XPEV shares are trading lower by 0.37% at $8.09 at the last check Tuesday.
Dislcaimer : This content was partially produced with the help of AI tools and was reviewed and published by Benzinga editors.
Photo via Shutterstock
© 2024 Benzinga.com. Benzinga does not provide investment advice. All rights reserved.
This article Future Of Travel: XPENG AEROHT Teams Up With Autoliv For Flying Car Safety Tech originally appeared on Benzinga.com .
- Credit cards
- View all credit cards
- Banking guide
- Loans guide
- Insurance guide
- Personal finance
- View all personal finance
- Small business
- Small business guide
- View all taxes
You’re our first priority. Every time.
We believe everyone should be able to make financial decisions with confidence. And while our site doesn’t feature every company or financial product available on the market, we’re proud that the guidance we offer, the information we provide and the tools we create are objective, independent, straightforward — and free.
So how do we make money? Our partners compensate us. This may influence which products we review and write about (and where those products appear on the site), but it in no way affects our recommendations or advice, which are grounded in thousands of hours of research. Our partners cannot pay us to guarantee favorable reviews of their products or services. Here is a list of our partners .
Can You Buy Travel Insurance for Road Trips?
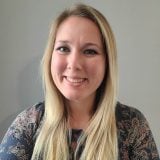
Many or all of the products featured here are from our partners who compensate us. This influences which products we write about and where and how the product appears on a page. However, this does not influence our evaluations. Our opinions are our own. Here is a list of our partners and here's how we make money .
Table of Contents
How road trip insurance works
How to choose between road trip travel insurance companies, best plans for road trip travel insurance, other tips for road trip travel insurance, top cards with travel insurance for road trips, road trip travel insurance recapped.
There’s nothing quite like breezing down a highway with the windows down, the wind in your hair and a cooler full of sodas in the back seat. Whether you’re traveling alone or enjoying a road trip with friends or family, you’ll want to be sure that you’re covered with travel protections on your drive. This is as true whether you're embarking on a classic American road trip — like U.S. Route 66 — are or braving the roadways in a foreign country, in your own car or a rented vehicle.
Let’s take a look at road trip travel insurance, how it works and the best plans for auto travel insurance to suit your needs.
Road trips aren't immune to disruptions, and road trip insurance works similarly to travel insurance . Most plans require purchase before your trip begins and cover your prepaid expenses.
For rental cars
Not all third-party travel insurance policies automatically include coverage for a rental vehicle. Seek out plans that provide rental car insurance specifically — or book your car with a credit card that offers these specific protections .
Rental car travel insurance policies generally protect against damage to the rental vehicle only. Causes not within your control, such as collision, theft, vandalism, windstorm, fire, hail or flood damage are typically covered.
Because of the limited scope of rental car insurance, know that you may be on the hook if you’re liable for damage to other vehicles or if there’s bodily injury.
When picking up your rental car, you will likely be offered the insurance sold by the rental car company at the counter, typically called a Collision Damage Waiver (CDW) or Loss Damage Waiver (LDW). You can decline this protection if you have sufficient coverage elsewhere (e.g., via a third-party policy or a credit card).
For your personal vehicle
Getting road trip insurance for your own vehicle isn't as common as travel insurance for rental cars , but it can still be a valuable consideration depending on your situation. For instance, access to roadside assistance can provide peace of mind if you are driving for long stretches in rural areas.
Finally, road tripping doesn’t end at driving a car. Other trip protections you may consider including in a travel insurance policy include medical evacuation, trip cancellation insurance, trip interruption coverage and accidental death and dismemberment (AD&D) insurance . This is true whether you opt to drive your own vehicle or rent one for your trip.
» Learn more: How to find the best travel insurance
With several travel insurance companies out there, it can be difficult to narrow down the one you really need. Before buying, always get quotes from multiple businesses.
This is because coverage levels and prices can vary dramatically. Insurance aggregators such as InsureMyTrip or Squaremouth (a NerdWallet partner) allow you to generate multiple quotes at once, plus they include filters for sorting so you can find the right policy for you.
Here are a few things to consider when looking for road trip insurance:
Whether you’re renting a car.
Where you're traveling.
Whether you already have car insurance.
What types of coverage you’re interested in.
If you’re also planning on flying, cruising or using a ferry.
How long you’ll be gone.
The cost of the plan.
To figure out the best road travel insurance, we combed a variety of insurance providers to check out their offerings.
Our quotes were built using a hypothetical 37-year-old from California taking a road trip throughout the United States. The total trip time was 15 days, and the total trip cost was $2,700. Here were the top contenders.
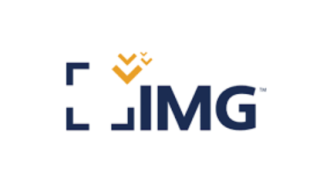
IMG’s iTravelInsured Travel SE plan came out significantly cheaper than competitors’ plans at just $100.43. This includes primary rental car coverage and a whole host of trip protections.
$40,000 in rental car coverage — $5,000 more than similar plans.
$250,000 in primary emergency medical coverage .
Pre-existing conditions covered.
Rental car coverage is only for collision, theft or damage to the vehicle.
$40,000 rental car coverage maximum.
AD&D doesn’t cover strokes or any cardiac events.
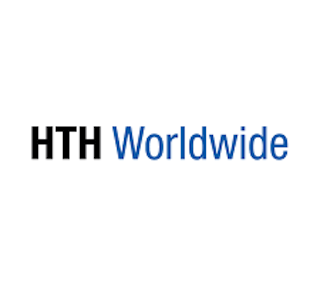
HTH Worldwide
HTH Worldwide is another top option for travel insurance for car trips. Coming in at $185.29, the TripProtector Preferred plan provides up to $35,000 for rental car coverage, as well as other unique coverage options.
Covers additional kennel fees for your pet if you’re delayed.
Includes $500,000 in primary emergency medical coverage.
Offers up to $200 to reinstate frequent traveler rewards, which is handy if you booked hotels on points for your trip.
Max of $35,000 in rental car coverage.
Repatriation of remains covers just $25,000.
Coverage excludes trucks, campers, motor bikes, recreational vehicles and exotic vehicles (defined as antique, limited production or collectible car or any other private passenger vehicle with a Manufacturer’s Suggested Retail Price over $75,000).
» Learn more: Is Cancel For Any Reason travel insurance worth it?
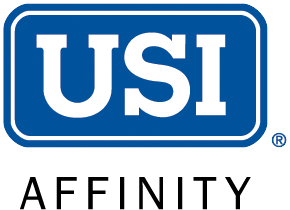
USI Affinity Travel Insurance Services
USI Affinity’s Diamond plan rang in at $187, just a little more than HTH Worldwide’s plan. While it includes rental car insurance, the limit is quite low, making this a better option for those who are driving their own vehicles.
Travel delay starting at six hours.
$1 million limit for medical evacuation and repatriation.
$50,000 limit for 24-hour AD&D.
$1,000 rental car insurance maximum.
$100 deductible for rental car insurance.
Maximum trip length of 30 days.
AXA Assistance USA
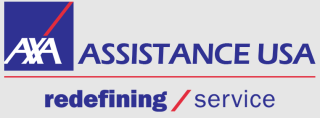
AXA Assistance USA is our final recommendation due to its nonmedical evacuation policy, which will transport you to safety during a natural disaster or time of political or civil unrest. This can be especially important if you plan on driving somewhere remote with unknown weather conditions. The total for AXA’s Gold plan came in at $215.
$50,000 in nonmedical evacuation.
$35,000 in rental car coverage.
Includes coverage for sporting equipment.
Minimum 12-hour travel delay before benefits kick in.
$25,000 limit for 24-hour AD&D.
Max of $35,000 in rental car coverage.
» Learn more: The best travel credit cards right now
We’ve gathered a few other tips for road trip travel insurance for you, so be sure to consider these before purchasing a policy:
Check out your own auto insurance policy. Within the U.S., your personal policy will usually also cover you when you drive a rental car.
Consider getting a travel credit card. Many travel credit cards include complimentary travel insurance as a benefit.
Secondary policies pay out after other options have been exhausted, but if you don’t have primary coverage, they automatically become primary. Keep this in mind when comparing plans.
Waive the rental company's insurance and pay for your car with one these travel cards to get complimentary insurance on your rental and more:
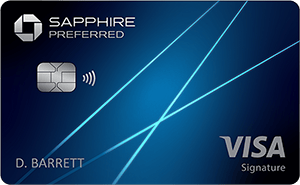
on Chase's website
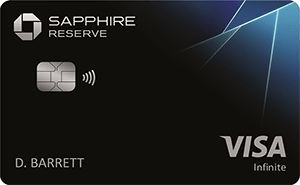
on American Express' website
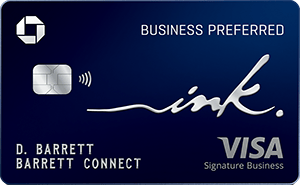
• Primary rental car coverage: Up to the cash value of most rental vehicles.
• Trip delay: Up to $500 per ticket for delays more than 12 hours.
• Trip cancellation: Up to $10,000 per person and $20,000 per trip. Maximum benefit of $40,000 per 12-month period.
• Trip interruption: Up to $10,000 per person and $20,000 per trip. Maximum benefit of $40,000 per 12-month period.
• Primary rental car coverage: Up to $75,000.
• Trip delay: Up to $500 per ticket for delays more than 6 hours.
• Secondary rental car coverage: Up to $75,000.
• Trip delay: Up to $500 per trip for delays more than 6 hours.
• Trip cancellation: Up to $10,000 per trip. Maximum benefit of $20,000 per 12-month period.
• Trip interruption: Up to $10,000 per trip. Maximum benefit of $20,000 per 12-month period.
Terms apply.
It’s important to ensure that you’re covered when you’re traveling, whether that’s by airplane, trains or automobiles. Depending on where you’re going, you may already have coverage — but do your due diligence and check first.
If not, consider getting a travel insurance policy that’ll cover your road trip, both for peace of mind and safety when you’re away from home.
American Express travel insurance disclosures
Insurance Benefit: Car Rental Loss & Damage Insurance
Car Rental Loss and Damage Insurance can provide coverage up to $75,000 for theft of or damage to most rental vehicles when you use your eligible Card to reserve and pay for the entire eligible vehicle rental and decline the collision damage waiver or similar option offered by the Commercial Car Rental Company. This product provides secondary coverage and does not include liability coverage. Not all vehicle types or rentals are covered. Geographic restrictions apply.
Eligibility and Benefit level varies by Card. Terms, Conditions and Limitations Apply.
Please visit americanexpress.com/benefitsguide for more details.
Underwritten by AMEX Assurance Company. Car Rental Loss or Damage Coverage is offered through American Express Travel Related Services Company, Inc.
Insurance Benefit: Trip Delay Insurance
Up to $500 per Covered Trip that is delayed for more than 6 hours; and 2 claims per Eligible Card per 12 consecutive month period.
Underwritten by New Hampshire Insurance Company, an AIG Company.
Insurance Benefit: Trip Cancellation and Interruption Insurance
The maximum benefit amount for Trip Cancellation and Interruption Insurance is $10,000 per Covered Trip and $20,000 per Eligible Card per 12 consecutive month period.
How to maximize your rewards
You want a travel credit card that prioritizes what’s important to you. Here are some of the best travel credit cards of 2024 :
Flexibility, point transfers and a large bonus: Chase Sapphire Preferred® Card
No annual fee: Bank of America® Travel Rewards credit card
Flat-rate travel rewards: Capital One Venture Rewards Credit Card
Bonus travel rewards and high-end perks: Chase Sapphire Reserve®
Luxury perks: The Platinum Card® from American Express
Business travelers: Ink Business Preferred® Credit Card
1x-10x Earn 5x total points on flights and 10x total points on hotels and car rentals when you purchase travel through Chase Travel℠ immediately after the first $300 is spent on travel purchases annually. Earn 3x points on other travel and dining & 1 point per $1 spent on all other purchases.
75,000 Earn 75,000 bonus points after you spend $4,000 on purchases in the first 3 months from account opening. That's $1,125 toward travel when you redeem through Chase Travel℠.
1x-5x 5x on travel purchased through Chase Travel℠, 3x on dining, select streaming services and online groceries, 2x on all other travel purchases, 1x on all other purchases.
75,000 Earn 75,000 bonus points after you spend $4,000 on purchases in the first 3 months from account opening. That's over $900 when you redeem through Chase Travel℠.
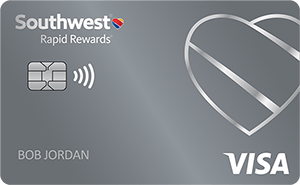
1x-2x Earn 2X points on Southwest® purchases. Earn 2X points on local transit and commuting, including rideshare. Earn 2X points on internet, cable, and phone services, and select streaming. Earn 1X points on all other purchases.
85,000 Earn 85,000 bonus points after spending $3,000 on purchases in the first 3 months from account opening.
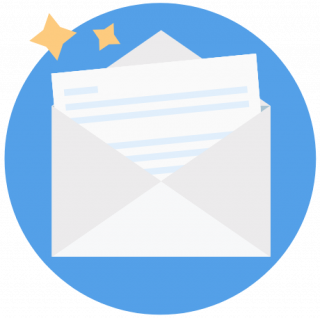

IMAGES
VIDEO
COMMENTS
You may review your total estimated reservation cost before you confirm your reservation. A non-refundable $25 application fee applies when you submit your Zipcar application. Zipcar is the world's leading car sharing alternative to car rental & ownership. Book cars on demand by the hour or day. Join instantly, drive in minutes.
BlaBlaCar is the world's leading community-based travel network. Bus or carpool, find the perfect ride from a wide range of destinations and routes at low prices. Choose your language. Čeština. Deutsch. English. English (India) Español. Español (Mexico) Français. Hrvatski. Magyar. Italiano. Nederlands.
Travelling by carpool is usually more affordable, especially for longer distances. Carpooling is also more eco-friendly, as sharing a car means there will be fewer cars on the road, and therefore fewer emissions. Taking a carpool ride is also a safe way to travel in the current times.
Enter a location and date and browse thousands of cars shared by local hosts. Book your trip. Book on the Turo app or online, choose a protection plan, and say hi to your host! ... Excellent car helped us travel all over Los Angeles, car in perfect condition! Chabe B. - Toyota Camry 2021. Great rental! Pansak W. - Toyota Yaris 2011. Good basic ...
Hitch Offers Private and Shared Ridesharing Between Cities. Easily Book Online or via the Mobile App. Door-to-door and Station-to-station Rides Available. Ride Drive Download Help Book Now. Rideshare for long distances. Rides between cities without compromise. Book your ride now.
Save time, money and hassle with local car rentals. Avail offers plenty of advantages compared to traditional car rental companies. When you borrow a vehicle with Avail, you won't have to worry about long lines or piles of paperwork. We guarantee consistency when you book with us. All of our locations are open every day from 5 am to midnight ...
Find your drive. Turo is the world's largest car sharing marketplace, where you can book the perfect car for wherever you're going from a vibrant community of trusted hosts across the US, UK, Canada, Australia, and France. Flying in from afar or looking for a car down the street, searching for a rugged truck or something smooth and swanky ...
Top 10 Car Sharing Services to Try. ZipCar — Top Pick. Getaround — Best Short-Term Bookings. Turo — Best Peer-to-Peer Car Sharing. Enterprise CarShare — Best for Businesses. HyreCar — Best for Gig Workers. Sixt — Best for Leasing. Urbi — Most Variety. GIG Car Share — Best for Eco-Friendly Rides.
You can request whatever you need from your Getaround rental car. Whether you're /going on a road trip with friends or taking a weekend getaway, Getaround car rental meets all your vehicle rental needs. Rent a car in an instant, open the car with the Getaround app, #1 for carsharing! 24/7. 200mi/day and insurance included.
Turo, Zipcar, Getaround, Maven, Enterprise CarShare, SHARE NOW, GIG Car Share, and Evo Car Share are popular services. Carsharing process includes booking through an app, making payments, picking up the car, and driving. The best car sharing services →. Car sharing rental process →. What is Carsharing - AKA "Airbnb for Cars"? 8 Best ...
Car sharing can complement these means of transportation. It works better in densely populated areas (ie. cities), it works better for people who have other ways to get around most of the time (ie ...
Rideshare for local carpool and long distance travel. Join a community of 150 000 members, connect with drivers & passengers to offer a ride or to book a seat. ... We were three friends riding with Karim from Toronto to Montreal and we all absolutely loved our shared travel time. Karim was on time for pick up, a reliable driver, and an ...
Introducing Uber's new and improved shared rides offering. With UberX Share, get everything you love about UberX at an even more affordable price. ... Help your city avoid extra emissions and car travel by sharing your ride. Designed to help keep you moving. ... The app will try to match your car with other riders heading your way. Get ...
How UberX Share works. 1. Request. Open the Uber app, enter your destination, and scroll down to the UberX Share ride option. 2. Ride. The app will try to match your car with other riders heading your way. Get additional savings if you're matched with a co-rider. See terms.*.
And fortunately for travelers, there are a wealth of new options for getting around using sharing economy resources. These fall into four distinct categories: Peer to peer car rentals. Membership based car rentals. Peer to peer taxi services. Peer to peer ride sharing. Peer to peer car rentals expand car rental options beyond the traditional ...
Earn up to 3,000 Nectar points when you share a lift and authenticate your journey in the Mobilityways app, as part of the Liftshare x Esso Thoughtful Driving Campaign. You'll earn 1,000 Nectar points for one trip authentication, plus an additional 2,000 Nectar points for 10 trip authentications made between 26th June and 2nd October 2024.
Simulation studies of shared autonomous vehicles have found that empty travel could increase the total vehicle travel distance by 10 to 100% in urban areas compared to the intended travel distance ...
Car sharing is so easy that we can safely say that it could be a great option for everyone! You don't even need to own a car, just search for drivers going the same way. Share traveling costs - significant annual savings for car owners and passengers. Meet new people - a great way to network. Reduce traffic - less congestion and air ...
When Evgeny Barkov owned a car, the 31-year-old software salesman would often look out of his Moscow window at it with disgust. His possession sat unused more than 90 percent of time, while ...
Within two years, the Moscow car-sharing system became one of the most developed shared mobility services in the world in terms of fleet and users' membership growth. On the other hand, Moscow's car owners still express the desire to use their private vehicles which, for many, remain a symbol of luxury and success (McKinsey 2018).
The impacts of ICT-based mobility services vary in different cities, depending on socioeconomic, urban form, and cultural parameters. The impacts of car-sharing and ridesourcing on public transport have not been investigated appropriately in post-Soviet Union cities. This study presents exploratory evidence on how ridesourcing and car-sharing affect public transport usage in Moscow ...
SGT Auto Transport: Best Guarantee. American Auto Shipping: Best Value. Sherpa Auto Transport: Best Pricing. Montway Auto Transport: Best Service. AmeriFreight: Best Discounts. The car shippers ...
Carsharing in Moscow. Carsharing in Moscow is a rapidly developing type of public transport. In January 2020, Moscow had the largest fleet of carsharing vehicles in the world, which counted more than 30,000 cars. The largest carsharing operator in Moscow is Yandex.Drive however, it is not available for foreign tourists.
Based on their shared commitment to future mobility safety, Autoliv and XPENG AEROHT will work together on various initiatives to develop safety solutions specifically for flying cars. "XPENG ...
IMG's iTravelInsured Travel SE plan came out significantly cheaper than competitors' plans at just $100.43. This includes primary rental car coverage and a whole host of trip protections. Pros ...
United Airlines - Airline Tickets, Travel Deals and Flights If you're seeing this message, that means JavaScript has been disabled on your browser, please enable JS ...