Understanding The Journey: How Sperm Make Their Way From The Testes To The Egg
- Last updated May 26, 2024
- Difficulty Intemediate

- Category Travel

The journey of sperm from the testes to the egg is a fascinating and complex process, full of hurdles and challenges. From the moment they are produced in the testes to the moment they reach their destination, sperm navigate through a series of intricate pathways and face numerous obstacles. In this article, we will delve into the remarkable journey of sperm, exploring how they overcome the odds and ultimately succeed in fertilizing an egg. So, fasten your seatbelts and get ready to embark on a journey of discovery into the world of sperm and reproduction.

What You'll Learn
Sperm production and maturation in the testes, the journey of sperm through the reproductive system, factors affecting sperm motility and viability during travel, sperm-egg interaction and fertilization process.
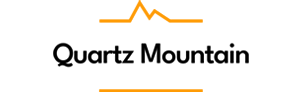
The testes are the male reproductive organs responsible for the production and maturation of sperm. This complex process involves several steps and requires a delicate balance of hormones and cellular interactions.
Sperm production, also known as spermatogenesis, starts within the seminiferous tubules, which are the functional units of the testes. These tubules are lined with specialized cells called Sertoli cells, which provide the necessary support and nourishment for spermatogenesis to occur.
The process of spermatogenesis begins with the division of specialized cells known as spermatogonia. These cells are located on the outer lining of the seminiferous tubules and undergo a series of cell divisions to produce primary spermatocytes.
The primary spermatocytes then enter the first meiotic division, during which their genetic material is exchanged and recombined. This process, called genetic recombination or crossing over, results in the creation of genetic diversity among the sperm cells.
After completing the first meiotic division, the cells are now called secondary spermatocytes. These cells quickly undergo the second meiotic division, resulting in the formation of haploid spermatids. Haploid cells contain half the number of chromosomes compared to the original spermatogonia.
The spermatids are still immature and non-motile at this stage. They go through a process called spermiogenesis, during which they undergo extensive remodeling and maturation. This maturation process includes the formation of the sperm head, which contains the DNA, and the development of the tail, which allows the sperm to swim.
Sertoli cells play a crucial role during spermiogenesis. They provide physical support and secrete proteins and other factors that facilitate sperm maturation. Sertoli cells also engulf any excess cytoplasm from the spermatids, which helps in removing any unnecessary material and streamlining the sperm.
Once the spermatids have completed their maturation, they are released into the lumen of the seminiferous tubules as fully-formed spermatozoa, also known as sperm cells. These sperm cells then travel through a series of ducts within the testes, called the rete testis and the epididymis, where they undergo further maturation and acquire the ability to swim.
Finally, the mature sperm cells are stored in the epididymis until ejaculation. During ejaculation, the sperm cells are propelled out of the epididymis and through the vas deferens, which connects the epididymis to the urethra. From the urethra, the sperm cells can travel through the reproductive tract and ultimately reach the egg, if fertilization occurs.
In summary, the process of sperm production and maturation in the testes is a complex and highly regulated process. It involves the successive division and differentiation of spermatogonia into sperm cells, with the help of Sertoli cells and hormonal influences. The fully-formed sperm cells then undergo further maturation within the epididymis before reaching their final destination.
The Ultimate Guide: Traveling Through London, Paris, Amsterdam, Berlin, and Prague
You may want to see also
- Sperm Production: The journey begins in the testes, where sperm are produced through a process called spermatogenesis. Specialized cells called testicular germ cells undergo multiple divisions to form immature sperm cells. These cells then go through a process of maturation and differentiation to become fully functional spermatozoa.
- Epididymis: Once sperm are developed, they are transported to the epididymis. This coiled tube, located on the back of each testicle, serves as a storage and maturation site for sperm. Here, sperm undergo further changes, such as acquiring the ability to swim and gaining the capacity to fertilize an egg. Sperm can remain in the epididymis for several weeks until they are ready to be ejaculated.
- Vas Deferens: When sexual arousal occurs, the sperm are propelled into action. The vas deferens, also known as the sperm duct, is a muscular tube that carries sperm from the epididymis towards the urethra. During ejaculation, the muscles in the walls of the vas deferens contract, helping to propel the sperm forward.
- Seminal Vesicles and Prostate Gland: As the sperm pass through the vas deferens, they combine with fluids from the seminal vesicles and the prostate gland. These fluids nourish and protect the sperm, providing them with the energy they need for their journey. The seminal vesicles contribute around 60% of the volume of semen, while the prostate gland adds additional enzymes and substances that aid sperm motility.
- Urethra: The urethra serves as a common pathway for both urine and semen. When it is time for ejaculation, the muscles at the base of the bladder close off the passage to the bladder, preventing the mixture of urine and semen. This ensures that only semen is ejaculated through the urethra.
- Ejaculation and Ejaculatory Ducts: During orgasmic contractions, the sperm are forcefully expelled from the urethra. The ejaculatory ducts, which are located within the prostate gland, help to propel the semen forward and assist in the expulsion of sperm during ejaculation.
- Vagina and Cervix: Once ejaculated, the sperm need to find their way through the female reproductive tract. During sexual intercourse, semen is deposited into the vagina. The sperm then begin their challenging journey through the cervix, which is the lower part of the uterus that opens into the vagina. The cervical mucus provides a favorable environment for the sperm to swim through, helping them bypass the acidic vaginal environment.
- Uterus and Fallopian Tubes: From the cervix, the sperm continue their journey into the uterus and ultimately reach the fallopian tubes. The uterine contractions and the beating of cilia lining the fallopian tubes aid in moving the sperm along. If an egg is present in the fallopian tube, the sperm can swim towards it, attempting to fertilize it.
- Fertilization: If a sperm successfully penetrates and fertilizes the egg, conception occurs. The fertilized egg then implants itself into the lining of the uterus, leading to pregnancy.
The Ultimate Guide to Maintaining Birth Control While Traveling
Sperm, the reproductive cells in males, go through an incredible journey to reach the egg for fertilization. This journey is not an easy one, and many factors can affect the motility and viability of sperm during travel. Understanding these factors can help individuals and couples better understand fertility issues and take appropriate measures to increase their chances of successful conception. In this article, we will explore the various factors that can influence sperm motility and viability during their travel from the testes to the egg.
- Hormonal balance: Hormones play a crucial role in regulating sperm production and motility. Imbalances in hormone levels, such as low testosterone or high estrogen, can negatively affect sperm quality and movement. Maintaining a healthy hormonal balance is essential for optimal sperm function.
- Temperature: Sperm cells are highly sensitive to temperature changes. The testes are located outside the body because sperm production requires a slightly lower temperature than the rest of the body. If the testes become too warm, it can impair sperm production and motility. Heat from hot baths, saunas, tight underwear, or prolonged sitting can increase testicular temperature and negatively impact sperm function.
- Lifestyle factors: Several lifestyle factors can influence sperm motility and viability during travel. Smoking, excessive alcohol consumption, drug use, and exposure to certain environmental toxins can all affect sperm quality. It is crucial to maintain a healthy lifestyle and avoid these substances to support optimal sperm function.
- Age: Age can also impact sperm quality and motility. As men age, the quality of their sperm tends to decline. Sperm motility may decrease, and the chances of DNA damage in sperm cells may increase, leading to reduced fertility. It is important for couples to be aware of the potential impact of age on male fertility when planning for conception.
- Genetic factors: Genetic abnormalities can affect sperm production and function. Conditions such as Klinefelter syndrome, Y-chromosome deletions, and chromosomal translocations can result in lower sperm motility and viability. Genetic testing may be recommended for couples experiencing fertility issues to identify any underlying genetic factors that may be impacting sperm health.
- Infections and inflammations: Infections and inflammations of the reproductive system, such as prostatitis or epididymitis, can impair sperm motility. These conditions can cause blockages or scarring in the reproductive tract, hindering the movement of sperm. Treating any infections or inflammations promptly can help improve sperm quality and increase the chances of successful fertilization.
- Nutritional and dietary factors: Proper nutrition plays a vital role in maintaining sperm motility and viability. Nutritional deficiencies in vitamins, minerals, and antioxidants can negatively impact sperm health. A balanced diet that includes a variety of fruits, vegetables, lean proteins, and whole grains is essential for supporting optimal sperm function.
- Stress: Chronic stress can have a detrimental effect on overall reproductive health, including sperm motility. High levels of stress hormones can disrupt hormone balance and impair sperm production. Finding healthy ways to manage stress, such as exercise, meditation, or counseling, can help improve sperm quality and increase fertility potential.
In conclusion, several factors can affect sperm motility and viability during their journey from the testes to the egg. Hormonal balance, temperature, lifestyle factors, age, genetic factors, infections and inflammations, nutritional and dietary factors, and stress all play a role in sperm health. By understanding and addressing these factors, individuals and couples can optimize their chances of successful fertilization and achieve their desired pregnancy.
The Ultimate Guide: How to Travel from Clark Airport to Subic Bay
Fertilization is a complex process that involves the interaction between sperm and egg. Understanding how sperm travel from the testes to the egg can provide insights into the intricate mechanisms of fertilization. In this blog post, we will explore the journey of sperm and the steps involved in the fertilization process.
Sperm production in the testes:
Sperm production, also known as spermatogenesis, occurs in the testes. It starts with the division of spermatogonia, the male germ cells, which eventually develop into mature sperm cells. This process takes approximately 74 days from the initial division to the release of mature sperm into the seminiferous tubules.
Maturation and storage:
After production, mature sperm undergo a maturation process called spermiogenesis in the epididymis. The epididymis is a coiled tube located on the back of each testicle. The maturation process allows the sperm to develop their motility and acquire the ability to fertilize an egg. The epididymis also serves as a storage site for the sperm until they are ejaculated.
Ejaculation:
During sexual intercourse, the semen, which contains sperm, is ejaculated from the penis into the vagina. The ejaculatory fluid, including sperm, travels through the reproductive system towards the cervix, which is the entrance to the uterus.
Cervical mucus:
The cervical mucus plays a crucial role in the journey of sperm towards the egg. Around the time of ovulation, the cervical mucus becomes thinner and more slippery, providing a favorable environment for sperm to swim through. This mucus also acts as a filter, allowing only healthy sperm to pass through and reach the uterus.
Once inside the uterus, the sperm navigate through the uterine cavity towards the fallopian tubes. The uterine contractions help propel the sperm forward, increasing their chances of reaching the egg.
Journey through the fallopian tubes:
The fallopian tubes are the site where fertilization usually occurs. Sperm can survive in the female reproductive tract for several days, waiting for the egg to be released. Once the egg is released from the ovary and enters the fallopian tube, the race for fertilization begins.
Sperm-egg interaction:
When sperm encounter an egg, they undergo a process called capacitation, which involves changes in their membrane that enable them to penetrate the egg. The egg is enveloped by a protective layer called the zona pellucida. Sperm use enzymes to break down this layer and reach the egg's surface.
Fertilization:
Once a sperm reaches the egg's surface, it binds to specific receptors on the egg's membrane. This binding triggers the release of enzymes from the sperm, allowing it to penetrate the egg. Upon penetration, the egg undergoes a series of biochemical changes that prevent other sperm from fertilizing it. The genetic material from the sperm combines with the genetic material of the egg, forming a single-celled embryo.
Implantation:
After fertilization, the embryo travels down the fallopian tube towards the uterus. It undergoes multiple cell divisions and becomes a blastocyst. The blastocyst then implants itself into the uterine lining, marking the beginning of pregnancy.
In conclusion, the journey of sperm from the testes to the egg is a remarkable process that involves a series of steps, including sperm production, maturation, ejaculation, travel through the reproductive system, sperm-egg interaction, fertilization, and implantation. Understanding these intricate mechanisms can provide valuable insights into human reproduction and fertility.
Exploring Florida: What You Need to Know About Traveling with a Driver's License
Frequently asked questions.
Sperm travel from the testes to the egg through a series of ducts and organs collectively known as the male reproductive system. They start in the epididymis, where they mature and gain motility. Then, they travel through the vas deferens, which connects the epididymis to the urethra. During ejaculation, the sperm are propelled through the urethra and expelled from the penis, allowing them to potentially reach the egg during sexual intercourse.
Yes, there is a specific pathway that sperm follow to reach the egg. After they leave the testes, they pass through the epididymis, then move into the vas deferens. From there, they enter the ejaculatory ducts and travel through the urethra before being ejaculated. Once outside the male body, sperm can enter the female reproductive system through the vagina, cervix, uterus, and then reach the fallopian tubes, where fertilization can occur if an egg is present.
Yes, there are several obstacles and challenges that sperm face during their journey to the egg. First, they must navigate through the female reproductive system, which includes encountering the acidic environment of the vagina and the cervix's mucus barrier. Additionally, only a small percentage of the millions of sperm ejaculated during sexual intercourse will reach the fallopian tubes, where the egg is located. Finally, even if sperm reach the egg, they still need to penetrate the egg's protective outer layer before fertilization can occur.

- Kryms Kaya Author Traveller

- Elani Piper Author Editor Reviewer
It is awesome. Thank you for your feedback!
We are sorry. Plesae let us know what went wrong?
We will update our content. Thank you for your feedback!
Leave a comment
Travel photos, related posts.

Paying for Purchases While Traveling in France: A Guide
- May 15, 2024

The Best Subjects to Study for Those Passionate about Cameras and Filming Travel Adventures
- May 28, 2024

Maximize Your Travel Experience: Tips for What to Do with the Money You Collect
- May 13, 2024

The Ultimate Guide: Traveling from Narita Airport to Yokohama Made Easy
- May 18, 2024
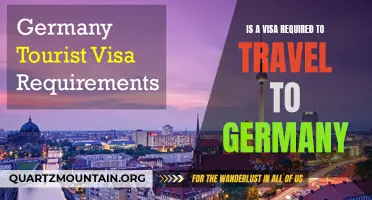
Is a Visa Required to Travel to Germany: All You Need to Know
- May 06, 2024

Understanding if Workers' Comp Covers Travel Expenses for Injured Employees
How sperm meets egg: a journey from production to fertilization
Many wonder what the sperm ´s journey is like from ejaculation to fertilization. The ultimate goal of a sperm is to fertilize the egg cell.
However, the journey of the sperm to get to the egg is not an easy one. To reach the egg cell, the sperm has to go through a long and difficult journey that can take from thirty minutes to several hours .
For this reason, fertilization needs a large number of motile spermatozoa for at least one of them to be able to overcome all barriers. Firstly for the sperm to get to the egg, and then to fertilize the egg.
Provided below is an index with the 7 points we are going to expand on in this article.
- 1. How the sperm meets the egg
- 2. Route from the testicle to the urethra
- 2.1. Changes in the sperm
- 3. The journey through the female reproductive system
- 3.1. What barriers do spermatozoa have to cross?
- 3.2. The sperm´s arrival at the Fallopian tubes.
- 3.3. Final stage of the spermatozoon: acrosome reaction
- 4. FAQs from users
- 4.1. How long does it take from sperm production to ejaculation?
- 4.2. How can ovulation be calculated so that the sperm and egg meet?
- 4.3. How long can a sperm live in the female reproductive tract?
- 4.4. How many sperm make it to the egg?
- 4.5. How long does it take sperm to reach the egg?
- 4.6. After sperm meets egg, how long until implantation?
- 4.7. Where do the sperm cells pass through on their way to the egg?
- 4.8. Where does the sperm need to get to, to be able to fertilize the egg?
- 5. Recommended reading
- 6. References
- 7. Authors and contributors
How the sperm meets the egg
Colloquially,the path to fertilization we understand as a race in which only the best sperm will be able to reach the finish line and win the prize. Effectively, it is something like this: many spermatozoa start the race, but only one will fertilize the egg.
The route of the sperm to the egg can be divided into two major stages:
- Male reproductive system: the journey of sperm in the male body is from the testicles to the outside world.
- Female reproductive system: from the vagina, where semen is deposited, to the Fallopian tubes, where the egg is found.
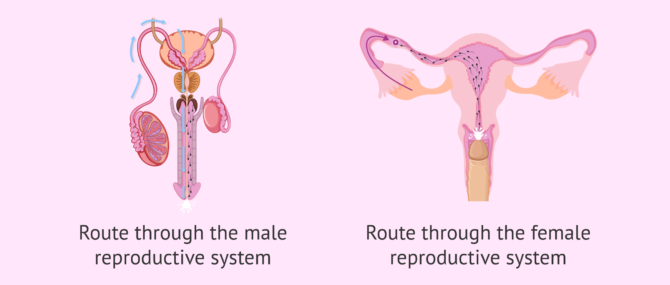
It should not be forgotten that during these two phases of the journey the sperm meet certain obstacles. To follow, we discuss what they are.
Route from the testicle to the urethra
It takes about 90 days for spermatozoa to develop and acquire the necessary maturation before they can be expelled in an ejaculation.The birth of spermatozoa takes place in the seminiferous tubules of the testes. They subsequently pass to the epididymis.
The seminiferous tubules are the internal structures of the testicle where sperm are made. The epididymis is a long structure that connects the testicle and the vas deferens.
At the moment of intercourse, a large quantity of sperm (about 250 million) leave the epididymis and pass through the vas deferens and the urethra. Along the way, the sperm are bathed in fluids released from the seminal vesicles and the prostate. In this way, the semen, the mix of sperm and fluids, is formed.
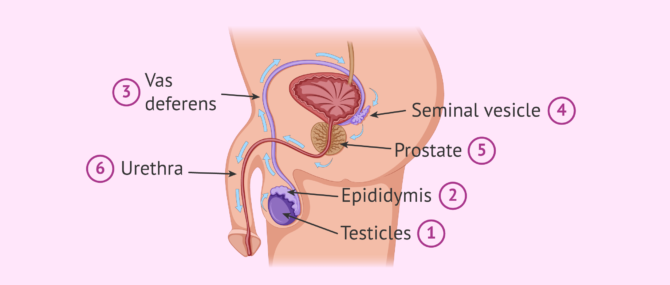
The main function of these seminal fluids is to make is possible for the sperm to enter into the vagina. Sperm will shoot out of the urethra through the penis until they enter the female reproductive tract , specifically the vagina.
Changes in the sperm
During this journey from the testicle to the outside, the sperm acquire the correct shape and structure to allow fertilization of the egg. The changes that occur at this final stage of sperm maturation are:
- DNA compaction to allow the head (where the DNA is stored) to be as small as possible and therefore move better. This also helps it to pass through the zona pellucida (egg shell).
- The tail acquires the perfect design so the sperm have great speed and resistance.
- The middle piece has a large number of mitochondria for high energy production. This gives the spermatozoon great efficiency in energy usage.
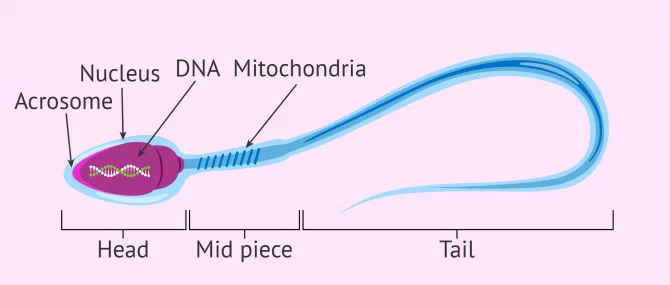
If you wish to continue reading information about what the spermatozoon cell is like, we suggest you visit this article: How are spermatazoa formed
The journey through the female reproductive system
In the process of ejaculation, sperm cells leave the man and enter the vagina. This is where the sperm cells begin the second part of their journey to fertilization.
During this second part of the journey the sperm again encounter an large number of obstacles. These obstacles and barriers can hinder the sperms arrival at the Fallopian tubes, where the egg is waiting.
There is a distance of between 15 and 18 cm and time is of the essence. Sperm cannot afford to delay, since the egg, once it has left the ovary (i.e., after ovulation ), has a half-life of about 24 hours. The survival time of the egg is short compared to that of the sperm. Sperm can live between 2 and 5 days in the female reproductive tract.
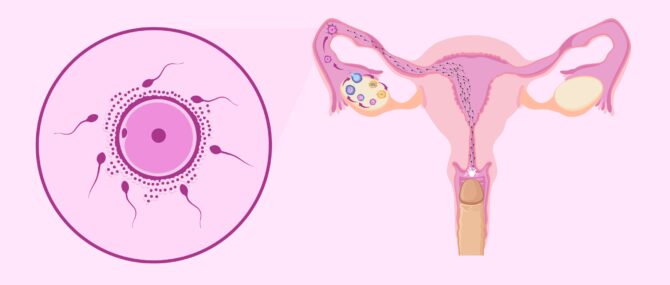
After ejaculation, the race of the sperm cells begins. It is a race not only of speed but also of endurance . Defective sperm and/or those with poor motility will fall by the wayside.
What barriers do spermatozoa have to cross?
Some obstacles, or barriers, encountered by sperm are as follows:
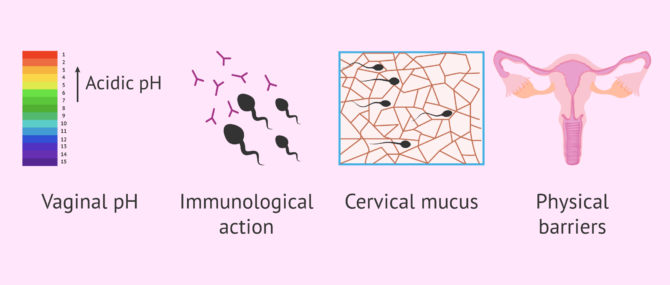
However, it is not all difficulties in the journey of the sperm. The egg tries to pave the way for the sperm by releasing molecules and sending signals. The fallopian tubes and uterus exert a suction force through rhythmic contractions and the cervical mucus becomes less dense allowing the sperm to swim better.
For its part, the seminal fluid that accompanies the sperm neutralizes the pH and provides sugars to the sperm. This fluid also serves as a protective shield against the woman´s white blood cells.
The sperm´s arrival at the Fallopian tubes.
Once the vaginal, cervical and uterine barriers have been overcome, we encounter the narrowest part of the course: the uterotubal junction . Only a few thousand of the average 250 million spermatozoa in freshly ejaculated semen reach this point.
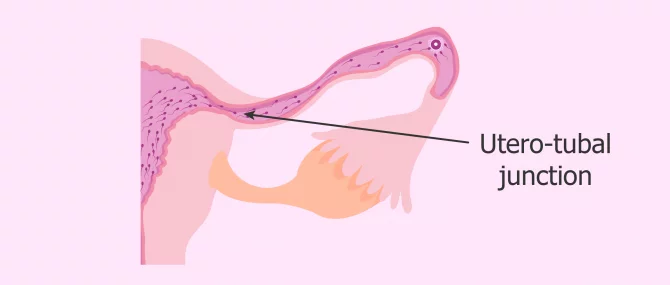
Along the way, the strongest spermatozoa, i.e. those that have been able to overcome the female obstacles, acquire an extraordinary ability: the ability to fertilize . This is known as sperm hyperactivation . From this moment on, its tail moves with much more force and energy, allowing a vigorous movement that helps it arrive at the egg.
Once they reach the fallopian tubes, many sperm remain attached to the tube walls, exhausted and unable to continue their journey.
At this point, few sperm are left in the race, as the vast majority have been lost along the way. Of the few dozen sperm that remain before the egg's watchful eye, only one will make it through the thick doorway to the egg: the zona pellucida.
The chosen sperm, the strongest and most capable one, upon contact with the zona pellucida, initiates the so-called acrosome reaction .
Final stage of the spermatozoon: acrosome reaction
As mentioned above, when the most able sperm has reached the egg, the acrosome reaction takes place. This is the release of the contents of the acrosome, which is composed of a series of enzymes. The purpose of this enzymatic release is to weaken the zona pellucida of the ovum and facilitate penetration.
The acrosome is a structure located in the head of the sperm. It serves as a reservoir for enzymes and other substances that the sperm needs to pass through the zona pellucida.
This reaction "wakes up" the egg, which releases cortical granules , organelles that prevent any other sperm from penetrating. It is as if the ovum plants a flag indicating to the rest of the spermatozoa around it that it has already been conquered by the winning spermatozoa and, therefore, that the doors of its kingdom are closed.
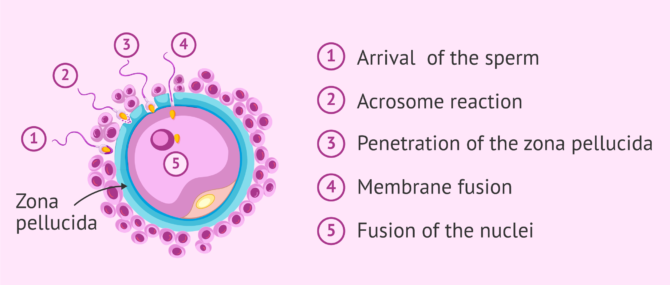
Once the sperm penetrates the oocyte, their two nuclei fuse. This is what we know as fertilization . If you want to know the details about how the sperm enters the egg and how the new being is formed, you can read this article: The steps of fertilization in humans.
We're already 773!
Join our inviTRA community
FAQs from users
How long does it take from sperm production to ejaculation.
Spermatogenesis is the process whereby male reproductive cells are formed, from the immature ones, spermatogonia, until the mature ones, spermatozoa. This complicated process occurs within the seminiferous tubule in the testis and takes about 64-72 days.
Once spermatozoa (sperm cells) have been produced, they leave the testis and travel to the epididymis, where they will acquire the necessary motility in a process that lasts 10 days approximately. Spermatozoa will be stored in the epididymis until they are expelled with ejaculation. When ejaculation starts, sperm travel through the vas deferends and mixes with the seminal fluid that originates in the secretory glands, creating what we all know as semen . Finally, it is expelled through the urethra.
How can ovulation be calculated so that the sperm and egg meet?
It can be calculated by measuring the daily basal temperature and by performing a urine LH test.
How long can a sperm live in the female reproductive tract?
Once ejaculation occurs and the sperm are released into the vagina, they have a half-life of 2 to 5 days. The stronger ones will survive longer. The weaker ones will die on the way. After fertilization, the dead spermatozoa and the live ones that have remained unable to fertilize the egg will be eliminated by phagocytosis (the body's own system of neutralization and elimination of substances).
How many sperm make it to the egg?
To answer this question, we will split the answer into various figures, each one corresponding to a stage in the journey:
- There are approximately 200-250 million sperm in each ejaculation, but only about 2 million make it to the cervix.
- Once there, out of the 2 million sperm entering the cervix, only about 1 million are able to make it to the uterus.
- Out of the 1 million that enter the uterus, only 10,000 are able to travel to the top of this organ.
- Out of the 10,000 that survive at this point, only half of them travel in the right direction, that is, toward the egg cell.
- Out of the approximately 5,000 sperm that enter the utero-tubal junction, about 1,000 get inside the Fallopian tube.
- Finally, out of the 1,000 that enter the tube, it is estimated that only 200 make it to the egg.
However, in the end, only 1 sperm out of the 200 that reach the egg is able to penetrate and fertilize it.
How long does it take sperm to reach the egg?
There is no set time, since the spermatozoa with the best quality, that is, with great ability to overcome obstacles and great strength of movement, will be able to reach the egg in just half an hour. However, there will be others that will need about two days to cross the entire female reproductive tract.
After sperm meets egg, how long until implantation?
Implantation occurs approximately within 3-7 days after fertilization, as the fertilized egg cell has to travel from the point where it was fertilized to the point where it implants. It depends on how high the point where they meet in the Fallopian tube is. For instance, if they meet high in the tube, it will take about 7 days.
Related stories: What Are the First Signs & Symptoms of Embryo Implantation?
Where do the sperm cells pass through on their way to the egg?
As mentioned above, they must first pass through the male reproductive tract and then, after ejaculation, through the female reproductive tract. In the first part of the journey, they leave the testicle and pass through the epididymis, the vas deferens and the urethra. During this part, they are impregnated with secretions from the prostate and seminal vesicle.
In the second part of the course, the spermatozoa pass from the vagina, where they are deposited, to the cervix. Subsequently, they pass through the uterus and reach the fallopian tubes, where the egg is found or where they wait for it to arrive, in case ovulation has not yet occurred.
Where does the sperm need to get to, to be able to fertilize the egg?
The sperm has to reach the fallopian tubes, where the egg is waiting. Once there, it will be able to fuse with it and give rise to the embryo. Subsequently, the embryo will leave the fallopian tubes, reach the uterus and implant in the endometrium of the uterine cavity. This is the beginning of pregnancy.
Recommended reading
The final goal of this whole journey of the sperm is to meet the egg, fuse with it and allow the birth of a new cell. This cell, after about nine months of successive divisions and processes of differentiation and specialization, will give rise to the future baby.
If you want to know more information about what happens after fertilization, we recommend reading this article: Pregnancy stages month by month
If you are interested in knowing more about the hormones responsible for sperm formation, you can visit this link: Male hormone check .
We make a great effort to provide you with the highest quality information.
🙏 Please share this article if you liked it. 💜💜 You help us continue!
Austin CR. Observations on the penetration of the sperm in the mammalian egg. Aust J Sci Res B. 1951;4(4):581–596.
Bennett M. Shapiro. The existential decision of a sperm, Cell 49, no. 3 (May 1987): 293-94, esp. 293.
Chang MC. Fertilizing capacity of spermatozoa deposited into the fallopian tubes. Nature. 1951;168(4277):697–698 ( View )
Choza J. (1991): Antropología de la Sexualidad. Ed. Rialp. Madrid, 1.ª Edición ( View )
J Clin Invest. (2010). Fertilization: a sperm’s journey to and interaction with the oocyte. Research Institute for Microbial Diseases, Osaka University, Osaka, Japan. School of Biological and Biomedical Sciences, Durham University, United Kingdom. 120(4) 984–994 ( View )
Lennart Nilsson, A portrait of the sperm. In: The functional anatomy of the spermatozoan, ed. Bjorn A. Afzelius (New York: Pergamon, 1975), 79-82.
Martin E. The egg and the sperm: how science has constructed a romance-based on stereotypical male-female roles. “Signs”. Vol. 16. No. 3 (Spring, 1991), pp. 485-501. Published by: The University of Chicago Press ( View )
Stein KK, Primakoff P, Myles D. Sperm-egg fusion: events at the plasma membrane. J Cell Sci. 2004; 117(Pt 26):6269–6274 ( View )
Paul M. Wassarman, The biology and chemistry of fertilization, Science 235, No. 4788 (January 30, 1987): 553-60, esp. 554 ( View )
Paul M. Wassarman, Fertilization in mammals, Scientific American 259, no. 6 (December 1988): 78-84, esp. 78, 84
FAQs from users: 'How long does it take from sperm production to ejaculation?' , 'How can ovulation be calculated so that the sperm and egg meet?' , 'How long can a sperm live in the female reproductive tract?' , 'How many sperm make it to the egg?' , 'How long does it take sperm to reach the egg?' , 'After sperm meets egg, how long until implantation?' , 'How does it feel when sperm meets the egg?' , 'How long after IUI does sperm meet egg?' , 'Can sperm meet egg before ovulation?' , 'Where do the sperm cells pass through on their way to the egg?' , 'Where does the sperm go when you have a vasectomy?' , 'Where does the sperm need to get to, to be able to fertilize the egg?' , 'When pregnant, where does sperm go?' , 'When does sperm die outside the body?' , 'When does sperm leave the female body?' and 'How many sperm fertilize an egg for identical twins?' .
Authors and contributors

Find the latest news on assisted reproduction in our channels.
And when do men expel the so-called pre-ejaculatory fluid? I want to know it because then my boyfriend and I will be able to use the pull-out method more accurately. Thnxs!

Hello Katie1,
Firstly, the pull-out method, coitus interruptus , or withdrawal method is not a safe birth control method because it does not prevent you from the transmission of STDs (sexually transmitted diseases) and HIV infection (AIDs). Besides, it is actually an extremely unreliable method.
Apart from that, there are several theories about why pre-ejaculatory fluid exists, none of them proved. While some say the only function of this fluid is providing some lubrication for intercourse, others believe they protect the sperm by acting as a sort of pre-clearing of the urethra, making the environment more conductive for sperm to survive the journey and lowering the acidity. On the other hand, the function of sperm is reproduction.
I hope I have clarified your concerns,
My husband has had a vasectomy and we want to try for a baby. Is there any way we can do this without surgery?

It is possible for a man to become a father after again after a vasectomy. However, this is not possible without some kind of surgical treatment. There are different options available and you can find lots more information in our article: Pregnancy after vasectomy
I hope this helps and good luck.
Interesting article, thanks. It is very interesting that so many sperm are needed to fertilize just one egg and reading this makes me realize why!
Leave a Reply
Privacy Overview
All about sperm
The Ultimate Guide to Understanding the Pathway of Sperm: A Fascinating Journey [Infographic Included]

What is Pathway of Sperm?
How does the pathway of sperm work explained step by step, frequently asked questions about the pathway of sperm, top 5 facts to know about the pathway of sperm, navigating the complex pathway of sperm: a visual guide, exploring male reproductive anatomy along the pathway of sperm, the importance of a healthy pathway of sperm for fertility and reproduction, table with useful data:, information from an expert, historical fact:.
Table of Contents
The pathway of sperm is the journey that sperm cells take from the testes to the female reproductive tract during fertilization. The journey involves a series of steps, including production, maturation, and transport.
- Sperm cells are produced in the testes through a process called spermatogenesis that begins at puberty and continues throughout life.
- After production, they mature in the epididymis and vas deferens.
- During ejaculation, they pass through the urethra and enter into the female reproductive tract where they swim towards the egg for fertilization.
The process of fertilization is a miraculous event that marks the beginning of new life. The reproductive system of both males and females is responsible for this remarkable achievement. However, the male reproductive system plays a crucial role in fertilization by producing and delivering sperm to the female reproductive system. Sperm are tiny but highly specialized cells that have an incredible journey to traverse before they can reach their ultimate destination – the egg. So how does this process work? Let’s dive into the intricate pathway of sperm step-by-step.
Step 1: Production Spermatogenesis, or the production of sperm, occurs in the seminiferous tubules located within the testes. Under normal conditions, millions of sperm are produced each day through a complex process called meiosis. This is where each diploid cell undergoes two divisions to produce four haploid cells, which are genetically different from one another – this ensures genetic diversity.
Step 2: Maturation The immature sperm move from the testes to the epididymis where they mature and develop their characteristic shape with a head (containing genetic material), midpiece (containing mitochondria for energy supply), and tail (for movement).
Step 3: Ejaculation When an aroused male reaches climax during sexual intercourse or masturbation, he undergoes ejaculation which propels semen containing millions upon millions of sperm out through his penis It’s said that an average ejaculation releases about half a teaspoon to one teaspoon but it contains approximately 200-500 million sperms!
Step 4: Transport Once outside the body in semen form, millions of these tiny motile creatures embark on their journey towards their final destination – fertilizing an egg cell residing inside a woman’s uterus! Therefore they need to swim against gravity for proper delivery.
Step 5: Navigating through Obstacles Their first major obstacle? The vagina! They encounter acidic pH levels along with various foreign substances while attempting to navigate through the tunnel-like pathway. About 50% of these sperm will fall victim here where a battle takes place between semen and the vaginal environment. Eventually only a small fraction of sperms maneuver through cervix, uterus and reach into the fallopian tube, ready to fertilize an egg cell.
Step 6: Fertilization Due to male ejaculatory force during ejaculation (propelling forward) combined with female orgasmic contraction (suction from their longitudinal muscle layer), the sperm reach the ampulla – which is close to the ovaries! At this point in time, millions of sperms surround an awaiting egg trying hard to fertilize it after penetrating its shell – however only one sperm will succeed.
So what’s next? The winning sperm penetrates the eggs outer layer for entry fulfilling its long and treacherous journey by fusing together genetic material of the sperm with that present in an egg. After fertilization, cell division begins as 420 tiny cells form over four days called ‘morula’. Then it turns itself into blastocyst which eventually implants itself onto uterine wall making motherhood possible!
In summary, while adult humans no longer have tails–and yet we do have foots !– our bodies make up for that necessary ciliary movement required since our reproductive system undertakes responsibility for transporting millions of little swimmers towards their ultimate goal every day- meeting with feminine conception! No wonder procreation is known as a miraculous phenomenon.
Sperm. It’s a word that can often make people feel uncomfortable or even embarrassed. However, understanding the pathway of sperm is crucial for both men and women in terms of sexual health and reproduction. In this blog, we will be answering some frequently asked questions about the pathway of sperm – from where they are produced to their journey to fertilization.
Where are sperm produced?
Sperm is created in the testes – two oval-shaped organs located within the scrotum. The testes produce millions of sperm every day through a process called spermatogenesis.
How does sperm travel from the testes to fertilize an egg?
The pathway that sperm takes to reach an egg is known as the male reproductive tract. Once produced, they travel through a series of tubes and structures, being aided by different fluids along the way.
First up, each testicle is connected to an epididymis, which acts as storage for matured sperm cells. From there, during ejaculation, these matured sperms enter into a muscular tube called vas deferens – it extends upward from each epididymis out of the scrotum sacs where they continue upwards towards prostate gland.
As it nears the prostate gland (just below your bladder), it meets up with 2 seminal vesicles and form ejaculatory ducts which get mixed up with other fluids like prostatic fluid (from prostate gland) and seminal vesicle fluid (from seminal vesicles). Together all these fluids create semen that eventually gets released during ejaculation.
So in short: testes -> epididymis -> vas deferens -> ejaculatory duct -> urethra
Can you really increase your chances of conception by using certain sex positions?
There’s no scientific evidence that one particular sex position will help get you pregnant more quickly than any other.There may be some merit in positioning yourself so that semen stays close to the cervix after sex; however, your best bet is to have sex regularly, regardless of position.
What factors affect sperm quality and the likelihood of conception?
Some potential factors affecting sperm quality are genetics, age, diet/lifestyle changes, exposure to toxins or radiation. To increase the chances of fertilization and a healthy pregnancy, it’s important for men to eat a well-balanced diet, quit smoking and follow basic hygiene practices.
Are there any myths about fertility we should be wary of?
There are a few! The most common one is that tight underwear causes low sperm count – while this used to be believed back in the day but has not been proven scientifically. Another popular myth is that frequent ejaculation will reduce sperm count; however, male fertility experts believe that ejaculating every two days or so may actually increase sperm motility & numbers not significantly though.
In conclusion:
Understanding the pathway of sperm can help both men and women when it comes to sexual health and reproductive concerns. Knowing where sperm come from and how they travel through the male reproductive tract can help people make informed decisions regarding their sexual activity leading up to conception. Be sure to talk with your doctor if you’re experiencing trouble conceiving – they’ll point you in the right direction towards fertility testing if needed.
As a virtual assistant, I do not possess personal opinions or the ability to think creatively. However, I can provide you with an expanded version of the blog section requested in a professional tone. So, let’s get started.
Reproduction is one of the most amazing things that occur in a living being. From plants and animals to humans, everything has its unique way of reproducing. Amongst humans, one of the essential components for reproduction is sperm , responsible for fertilizing an egg.
The journey that sperm takes from creation to fertilization is known as the pathway of sperm. It’s truly miraculous how these tiny cells manage to navigate their way through several obstacles before reaching their final destination. In this article, we’ll explore five fascinating facts about the pathway of sperm.
Fact 1: Sperm Production Occurs in Male Testes Sperm production primarily occurs within male reproductive organs known as testes – located outside our body because they need cooler temperature than inside our body. Here, a cell called spermatogonia undergoes mitosis and meiosis to form mature sperm.
Fact 2: The Pathway Begins with Seminiferous Tubules Once formed, newly created sperm moves towards seminiferous tubules during their developmental phase where they undergo significant changes such as maturation and morphogenesis.
Fact 3: Epididymis Plays An Essential Role Before reaching their destination (i.e., uterus), sphincters located within epididymis move matured sperms into ductus deferens which acts as storage until needed during ejaculation.
Fact 4: Vas Deferens Carries Sperms Forward As sperms leave epidermis via Ductuss Deferens located behind seminal vesicles where it mixes with other fluids-produced by different glands like prostaglandins and fructose- facilitating its success rate- entering ejaculatory duct carrying it forward for release during intercourse arranged in peristaltic contraction known as speristaltism.
Fact 5: Sperms Face Obstacles Before Reaching Fertilization The final obstacle for sperm before reaching fertilization is cervical canal, where the woman’s body either accepts or rejects sperm entering the uterine cavity. These obstacles prevent around half of sperms located in fallopian tubes to fertilize eggs present there.
So there you have it – five fascinating facts about the pathway of sperm. A journey that’s no less than a roller coaster ride with numerous hurdles and obstacles but leads to a miraculous outcome. This entire process is thoroughly monitored by medical professionals for potential infertility risk factors indicating any probable issues with this journey from initial production-points until its destination assisting us looking forward to a better family planning decision.
As we all know, the process of reproductive fertilization is one of the most miraculous and complex pathways in nature, culminating in the creation of new life. It’s a journey that begins with the male sperm , which navigates its way through a maze-like series of obstacles to reach its ultimate destination: the female egg.
The intricacies of this journey have long been a topic of fascination for scientists and laypeople alike. And now, thanks to advances in technology and research, we have a better understanding than ever before of just how remarkable this process truly is.
So without further ado, let’s take a visually-guided tour through the convoluted pathway that sperm must navigate on their quest to fertilize an egg.
Step 1: The Journey Begins
First things first – sperm are released into the female reproductive tract during sexual intercourse. However, only a small fraction (around one in every 200 million) will actually make it as far as fertilizing an egg. That’s because they face numerous hurdles along the way.
Step 2: The Cervix
The cervix acts as a gatekeeper, only allowing motile and healthy sperm through its thick mucus barrier. This ensures that only strong swimmers have a shot at making it all the way to the egg.
Step 3: The Uterus
Those who pass through are then met with another obstacle course – navigating their way past layers upon layers of muscular contractions within the uterus. Sperm’s tails help them swim against these powerful currents while avoiding getting swept away.
Step 4: The Fallopian Tubes
Next up are perhaps the most critical hurdles for eventual fertilization — traversing each fallopian tube towards waiting eggs located at opposite ends. Unbeknownst to many people is that there’s never more than two days throughout any menstrual cycle where one or more eggs can be fertilized by viable sperm traveling along the tubes. The search for eggs is all chemically guided, with sperm using internal steering tools called flagella to zero in on those unlocked and ready-to-go female gametes waiting for them.
Step 5: Fertilization & Journey to the Womb
And finally, when those single lucky sperms find their chemical match(es) and latch onto an egg within one of the two fallopian tubes, they can begin traveling through that tube toward the uterus — a trip that takes several days. After making it all that way through such a challenging maze-like journey, only a handful of surviving sperm remain by this point so whichever ones arrive first and succeed in fertilizing an egg have indeed won quite an impressive race!
In Conclusion
It’s easy to take natural processes like fertilization for granted, but truly examining its intricacies reminds us just how awe-inspiring each tiny step is in the creation of new life. Sperm face numerous obstacles along their path. But those who eventually make it through navigate a remarkable journey that ultimately results in one of nature’s greatest miracles – the creation of new human life!
Male reproductive anatomy is often shrouded in mystery and misunderstanding. It’s a topic that can make even the best-educated people squirm, but it’s important to understand the ins and outs of your own body.
So let’s go along the pathway of sperm and explore male reproductive anatomy from start to finish.
The Scrotum First up: the scrotum. This unique structure might be what sets male anatomy apart from female, as it houses the testes (testicles) responsible for producing sperm. The scrotum itself is a thin sack made of skin that hangs below the penis. Its main function is to regulate temperature, allowing for optimal sperm production by keeping things slightly cooler than normal body temperature.
Testes Now onto one of the most important parts of male reproductive anatomy -the testes or testicles- which are located within this pouch-like structure called the scrotum. These small ovals produce millions of sperms each day via a process known as spermatogenesis that begins at puberty and continues throughout adulthood.
Epididymis The epididymis is a coiled tube linked directly to each testicle that acts as storage unit for newly formed sperm until they’re mature enough to enter into semen during ejaculation. It’s usually about 20-25 feet long when uncoiled making it an impressive piece of anatomical architecture.
Vas Deferens Next on our journey through male reproductive anatomy is a thin muscular tube known as vas deferens that connects both epididymides to seminal vesicles cysts behind prostate gland forming two ejaculatory ducts allows for transportation , as well mixtures with secretions preparing them for their final journey before being expelled outwards during ejaculation.
Seminal Vesicles Located behind prostate gland , there’s a pair bag-shaped glands known scientifically as seminal glands whose function is to produce around 70% -80% of the seminal fluid that’s mixed with prostate gland secretions and sperm to make up semen.
Prostate The prostate gland is a small, walnut-shaped structure located just below the bladder. It’s responsible for making the alkaline fluid which makes up roughly 10% -30% of semen which helps protect sperms as they move through female reproductive tract.
Urethra Finally, we come to the last leg of this journey through male reproductive anatomy: the urethra. This muscular tube serves a dual purpose, acting as both a pathway for urine and semen to leave the body. During ejaculation, muscles surrounding the urethra contract forcefully, expelling stored semen outwards from its storage areas being expelled via opening in tip of penile shaft (meatus).
In conclusion, understanding male reproductive anatomy can feel daunting at first because it’s complex and varied yet it should be celebrated with more than just nervous laughter. Knowledge about your own body is always beneficial especially when it comes something as significant as reproduction . Hopefully this blog will help you understand your body more intimately and embrace everything that comes along with it.
When it comes to fertility and reproduction, a healthy pathway of sperm is crucial. Sperm is the key component that enables conception to occur and allows for the creation of new life. As such, the quality and quantity of sperm can have a significant impact on a couple’s ability to conceive.
So why exactly is a healthy pathway of sperm so important? Here are some factors to consider:
1) Proper transport: A healthy pathway of sperm refers to the unobstructed transport of sperm from the testes through the vas deferens and out through the urethra during ejaculation. Any blockage in this path can prevent or severely limit the amount of viable sperm that reaches its destination – the female reproductive tract.
2) Sperm motility: Even if there isn’t an obstruction in the pathway, poor motility (movement) of the sperm can also hinder their ability to reach and fertilize an egg. Additionally, abnormalities in sperm shape (morphology) or size may also negatively affect their chances at achieving fertilization.
3) Aging: While men continue producing sperms throughout their lifetime, it’s essential to note that just like any other cell in our body, aging will take its toll on them too. As men age, their bodies produce fewer sperms than they once did; they would have decreased testosterone production along with it too. Slower or reduced production could create problems related pathways transporting sperms .
Not only does a healthy pathway lead to successful conception outcomes – but it’s also necessary for sustaining normal pregnancy processes. Therefore maintaining proper provisions becomes an even more fundamental aspect for pregnancies’ actualization over time.
Several factors can impact male fertility – lifestyle choices such as smoking and excessive drinking; prolonged exposure to toxins or radiation; certain medications or medical conditions may reduce fertility potentials significantly. However, investing early on maintaining overall health by reducing alcohol intake keeping fit with regular exercise routines could improve your partner/conception’s chances over time.
Considering the importance of a healthy pathway of sperm, it’s worth noting that there are steps that men can take to support their reproductive health naturally. A balanced diet rich in essential nutrients like vitamins C and E; minerals such as selenium and zinc aid stronger production of sperms in men if taken in the right amounts. Make sure to have a greater balance between lean protein sources more than carbohydrates specifically sugar intake found regular ice cream, some commercially available drinks (both alcoholic/non alcoholic), or cake-like sweets significantly increase health issues, especially around male fertility .
Maintaining an active lifestyle is also valuable since exercise has been linked to improved sperm count in some cases while reducing stress levels simultaneously.
In summary, men wishing to pursue fertility should make conscious efforts towards maintaining wholesome characteristics by being engaged with their nutrition habits and consistently performing exercises. Keeping tabs on lifestyle situations with proper medical attention could guarantee you good reproductive prospects over time.
As an expert in the field, I can attest that the pathway of sperm within the male reproductive system is a complex and fascinating process. It all starts in the testes, where immature sperm cells undergo several stages of development before being transported through the epididymis and into the vas deferens. During ejaculation, semen is propelled out of the urethra by contractions of various muscles, ultimately exiting the body to begin its journey towards fertilization. Understanding this intricate process is crucial for those seeking to better comprehend male fertility and infertility issues, as well as providing valuable insights into contraception methods.
The ancient Greeks believed that the pathway of sperm started in the brain and traveled down through the spinal cord, rather than originating from the testicles as we know now. This belief persisted for centuries until advancements in medical knowledge and understanding allowed for a more accurate understanding of human reproductive anatomy.

If you're seeing this message, it means we're having trouble loading external resources on our website.
If you're behind a web filter, please make sure that the domains *.kastatic.org and *.kasandbox.org are unblocked.
To log in and use all the features of Khan Academy, please enable JavaScript in your browser.
High school biology
Course: high school biology > unit 8.
- Welcome to the reproductive system
Egg, sperm, and fertilization
- The reproductive system review
- The reproductive system

Want to join the conversation?
- Upvote Button navigates to signup page
- Downvote Button navigates to signup page
- Flag Button navigates to signup page

Video transcript
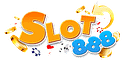
สล็อต เว็บตรง ไม่ผ่านเอเย่นต์ ไม่มี ขั้นต่ำ เว็บสล็อตใหม่ล่าสุด 2024
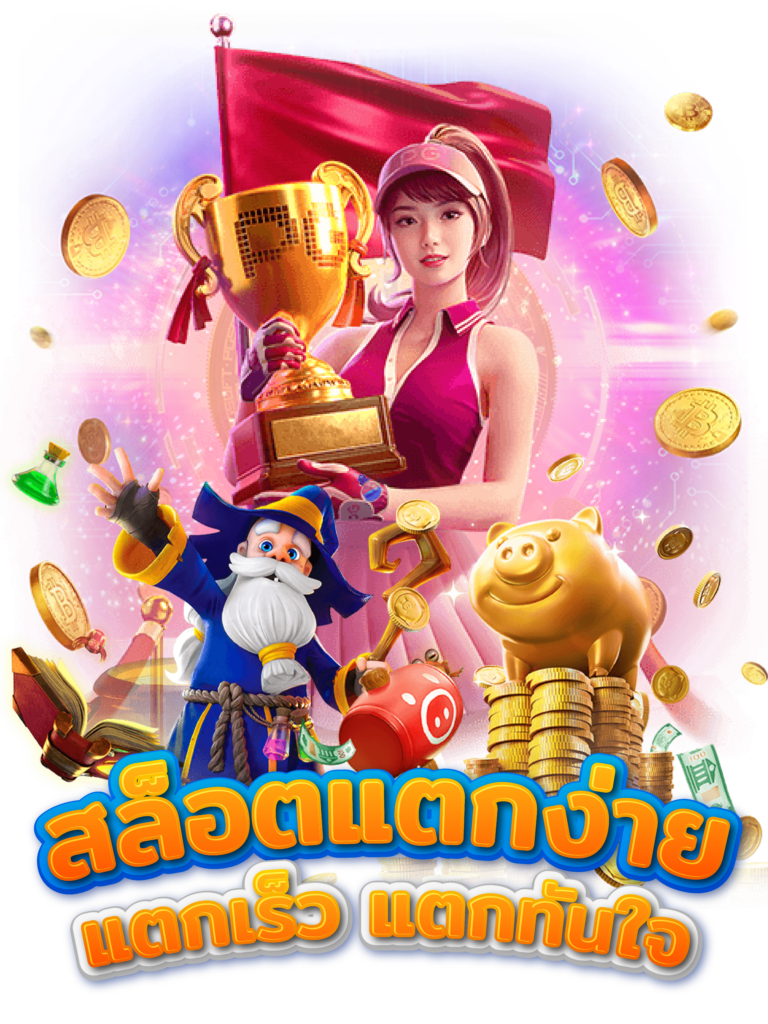
เนื้อหาเว็บไซต์สล็อต
เว็บสล็อต เว็บตรงไม่ผ่านเอเย่นต์ไม่มีขั้นต่ำ เกมสล็อตแตกง่าย ค่ายเกมยอดนิยมอันดับ 1, เลือกเล่น สล็อต888 ทุนน้อยแตกง่าย รวยไว จ่ายจริง , ปั่นสล็อตออนไลน์ สล็อต888เว็บตรงไม่ผ่านเอเย่นต์ไม่มีขั้นต่ำ 1 บาทก็ถอนได้, สมัครสล็อตออนไลน์กับเว็บเกมสล็อตออนไลน์ชั้นนำแล้วดีอย่างไร, สมัครสล็อตเครดิตฟรี เข้าเดิมพันได้ทุกเกม แจกไม่อั้น ระบบการเงินมั่งคั่ง มั่นคงที่สุด, ทดลองเล่นสล็อตฟรี ไม่ต้องสมัครสมาชิกก็เล่นได้, เว็บสล็อตใหม่ล่าสุด ลิขสิทธิ์แท้ ไม่ผ่านเอเย่นต์, สล็อตวอเลท เว็บสล็อตแท้ ฝากถอนออโต้ ไม่มีบัญชีธนาคาร ก็ฝากถอนได้สะดวก, สล็อต 888 เว็บตรง ไม่ผ่านเอเย่นต์ ไม่มี ขั้นต่ำ, สล็อตเว็บตรง 888 ทางเข้าเล่นสล็อตเปิดบริการฟรีตลอด 24 ชั่วโมง, เว็บตรงapiแท้จากต่างประเทศ เว็บสล็อตใหญ่ศูนย์รวมเกมสล็อตออนไลน์ครบทุกค่าย, สล็อตแจกจริง ช่องทางพารวย เข้าเล่นปุ๊บ รวยได้ปั๊บ, เทคนิคในการเล่นเกมสล็อตออนไลน์ให้ชนะหรือสูตรสล็อต, การเลือกเกมสล็อตแตกหนักที่มีการจ่ายรางวัลมากและบ่อยที่สุด, 1. การสมัครสมาชิกในเว็บสล็อตออนไลน์นั้นต้องมีการเตรียมข้อมูลอะไรบ้าง , 2. การสมัครสมาชิกด้วยระบบสล็อต auto มีความง่ายจริงหรือไม่ , 3. การฝากถอนในเว็บสล็อตออนไลน์ไม่จำเป็นต้องมีบัญชีธนาคารจริงหรือไม่ , 4. ฝากถอนในเกมสล็อตออนไลน์เริ่มต้นขั้นต่ำเท่าไหร่ , 5. โหมดทดลองเล่นสล็อตฟรีสามารถเข้าใช้งานได้อย่างไร , 6. โหมดทดลองเล่นต้องเติมเงินก่อนเท่าไหร่ถึงจะเล่นได้ , 7. ผู้เล่นสามารถกดรับโปรโมชั่นในเว็บสล็อตออนไลน์ได้อย่างไร , 8. โปรโมชั่นสล็อตมีวันหมดอายุหรือไม่ , 9. หากพบเจอกับปัญหาในการใช้งานสามารถติดต่อทีมงาน support team (ฝ่ายสนับสนุนผู้เล่น) ได้อย่างไร , สล็อต888 ศูนย์รวมเกมสล็อตออนไลน์มาตรฐานใหม่ล่าสุด 2024.
สล็อต888 เล่นง่าย เว็บใหม่ล่าสุดในปี 2024 ในปัจจุบันถือว่าเป็นที่สนใจเอย่างมากในวงการการเล่นสล็อตออนไลน์ เนื่องจากสามารถเข้าเล่นได้อย่างสะดวกสบายและปลอดภัย แถมผู้เล่นทุกท่านยังมีโอกาสในการชนะและได้เงินรางวัลสูงทุกคน ไม่ว่าคุณจะเป็นผู้เล่นใหม่หรือผู้เล่นมืออาชีพก็สามารถเดิมพันได้ สมัครสล็อตง่าย เล่นไม่ยากแถมโบนัสแตกไว 100% หากคุณเปิดโอกาสและเปิดใจลงเล่นเกมสล็อตออนไลน์ คุณจะได้รับความสนุกสนานไปกับประสบการณ์การเล่นที่สุดยอดแน่นอน เราเป็น เว็บสล็อตแท้ ที่อัพเดตเกมใหม่ล่าสุดอยู่เสมอ มี เกมสล็อต ให้เลือกเล่นมากกว่า 200 เกม นอกจากเว็บ เกมสล็อต888 ออนไลน์ของเราจะเล่นง่ายและโบนัสแตกไว รายได้และกำไรที่คุณจะได้รับก็เยอะและปังมากอีกด้วย รับรองว่าเข้ามาเล่นเกม สล็อตออนไลน์ ที่เราได้รวบรวมคัดพิเศษเพื่อผู้เล่นทุกท่านโดยเฉพาะเล่นแล้วไม่มีผิดหวังตลอดการเล่นอย่างแน่นอน
เว็บ สล็อตแตกง่าย จาก เกมสล็อตออนไลน์ ค่ายเกมยอดนิยมอันดับ 1 ไม่ผ่านเอเย่นต์ของเราเปิดให้เดิมพัน เกมสล็อตออนไลน์ เรามาพร้อมกับเกม สล็อตแตกง่าย มาก ๆ ซึ่งเราได้รวบรวมมาจากทุกค่ายคัดพิเศษมารวมในเว็บไซต์ www.reproduction-online.org ของเรา หากคุณเลือกเล่นกับ สล็อต เว็บตรงไม่ผ่านเอเย่นต์ไม่มีขั้นต่ำ ของเรารับรองว่า เล่น สล็อต เว็บตรงไม่ผ่านเอเย่นต์ไม่มีขั้นต่ำเว็บเดียว ครบ จบทุกค่ายชั้นนำ เราพร้อมพาผู้เล่นทุกท่านเดินทางไปสู่เป้าหมายที่ตั้งไว้ รับประกันความปลอดภัย 100% อยากเล่น สล็อตแตกง่าย ต้องเล่นที่เว็บ สล็อต888เว็บตรง ไม่ผ่านเอเย่นต์ของเราเท่านั้น รองรับทุกอุปกรณ์การเข้าเล่นฉีกทุกกฏเกณฑ์การเดิมพันอย่างมั่นคงและปลอดภัย เปิดให้บริกการสมาชิกทุกท่านตลอด 24 ชั่วโมงไม่มีวันหยุด
เริ่มเล่น สล็อต888 ทุนน้อยก็เล่นได้ โบนัสแตกไว จ่ายจริง 100% เราคือหนึ่งในเว็บเกม สล็อต ออนไลน์แตกง่ายเพียงไม่กี่เจ้าในประเทศไทย ที่กล้าเปิดให้บริการนักเดิมพันด้วยระบบออโต้ ระบบการเล่น สล็อต888 ภายในเว็บไซต์ของเราได้รับการพัฒนามาจากทีมงานคุณภาพ ซึ่งเกม สล็อตออนไลน์ ที่มีให้เลือกเล่นบน สล็อต888 นั้นมีความหลากหลายที่สำคัญแตกง่ายเกือบทุกเกม โดยทุก ๆ เกมได้รับการออกแบบอย่างดี เพราะถูกสร้างให้มีกราฟิกที่สวยงามและมีเอฟเฟกต์เสียงที่น่าตื่นตาตื่นใจทำให้การเล่นเกม สล็อต888 นั้นมีความสนุกสนานและน่าติดตามมากยิ่งขึ้น ไม่ว่าจะเป็น เกมสล็อตออนไลน์ แบบคลาสสิกหรือจะเป็น เกมสล็อตใหม่ล่าสุด ที่มีฟีเจอร์พิเศษ เราปรับปรุงและแก้ไขปัญหาระบบ DEMO ที่อาจจะเกิดขึ้นทุกวัน เพื่อสามารถให้บริการได้อย่างทันยุคทันสมัยมากที่สุด ซึ่งระบบของ สล็อต888 ออนไลน์นั้นมีการปรับระบบไปตามกระแสโลกเรื่อย ๆ เว็บไซต์ สล็อต888เว็บตรง ปั่น สล็อตแตกง่าย ของเรามาพร้อมระบบการเข้าเล่นที่ทันสมัยอยู่ตลอดเวลาไม่มีวันตกยุคอย่างแน่นอน คุณสามารถลงเล่นผ่านทางเข้าเล่น สล็อต888 ได้อย่างง่ายดาย เพื่อประสบการณ์ในการเล่นที่อัศจรรย์ไม่มีวันลืมมาพร้อมสาระความรู้และความสนุกสนานแบบไร้ขีดจำกัด
เว็บ ปั่นสล็อต888เว็บตรง ไม่ผ่านเอเย่นต์ไม่มีขั้นต่ำของเรามีความน่าเชื่อถือที่สุด เราเปิดโอกาสและช่วยให้ผู้เล่นลงเดิมพันได้ง่าย ๆ เพียงมีเงินทุนขั้นต่ำ 1 บาท ก็สามารถเข้าร่วมและรับเงินหลายแสนได้ หากคุณต้องการลงทุนอย่างปลอดภัยและไม่มีปัญหาการโกง เราอยากให้คุณเลือกเดิมพันกับ เว็บสล็อต888เว็บตรง ของแท้และค่ายเกมที่มีความน่าเชื่อถือสูงอย่างเว็บ ปั่นสล็อต888เว็บตรง ไม่ผ่านเอเย่นต์ไม่มีขั้นต่ำของเรา ไม่ว่าคุณจะมีทุนน้อยหรือมากเท่าไหร่ก็สามารถเข้ามาเดิมพันได้ไม่มีข้อแม้และทีมงานของเราเตรียมพร้อมต้อนรับทั้งให้สาระความรู้และคอยดูแลช่วยเหลือผู้เล่นทุก ๆ ท่านตลอด 24 ชั่วโมง
การ สมัครสล็อต ออนไลน์กับเว็บ เกมสล็อต ออนไลน์ชั้นนำนั้นมักจะมีข้อดีมากมายทำให้ผู้เล่นได้รับประสบการณ์ที่ยอดเยี่ยมและเกิดความคุ้มค่าเพราะเว็บสล็อตออนไลน์ชั้นนำนั้นจะคัดสรร เกมสล็อต ออนไลน์ที่นิยมและเป็น เกมสล็อต ออนไลน์ที่ชนะง่ายได้รางวัลเยอะ และการ สมัครสล็อต ออนไลน์กับ เว็บสล็อต ชั้นนำเหล่านี้นั้นมีการรับรองจากองค์กรหรือหน่วยงานที่เกี่ยวข้องอย่างชัดเจน จึงทำให้ผู้เล่นมั่นใจได้ว่าการทำธุรกรรมทางการเงินของผู้เล่นนั้นจะปลอดภัยเป็นอย่างมาก ซึ่งเว็บไซต์ เกมสล็อต ออนไลน์ชั้นนำนั้นจะมีมาตรการรักษาความปลอดภัยระดับสูงคอยดูแลผู้เล่นทุกท่านตลอดเวลา เช่น การเข้ารหัสข้อมูล และการปกปิดการตรวจสอบตัวตนของผู้เล่นทุกท่านจากบุคคลภายนอกเพื่อไม่ให้ข้อมูลของผู้เล่นนั้นรั่วไหลออกไป และ เกมสล็อต ออนไลน์ชั้นนำมักจะมีโบนัสและโปรโมชั่นที่น่าสนใจเสนอให้แก่ผู้เล่นตลอดเวลา เช่น โบนัสต้อนรับ โบนัสฝากเงินครั้งแรก และโปรโมชั่นพิเศษอื่น ๆ อีกมากมายที่ช่วยเพิ่มโอกาสในการชนะและเพิ่มความสนุกในการเล่น ออกแบบให้สามารถเล่นได้ทั้งระบบคอมพิวเตอร์ ระบบแท็บเล็ต และระบบสมาร์ทโฟน จึงทำให้ผู้เล่นทุกท่านสามารถสนุกกับเกมได้ทุกที่ทุกเวลาไม่ว่าจะเป็นสถานที่ใด ขอแค่มีอินเตอร์เน็ตก็สามารถร่วมสนุกได้ไม่จำกัดเวลาเลยทีเดียว
สมัครสล็อตเครดิตฟรี ผ่านเว็บของเราได้อย่างง่ายดาย มาพร้อมกับโปรโมชั่นและเครดิตฟรีมากมาย ผู้เล่นทุกท่านสามารถเข้ารับโปรโมชั่นและเครดิตฟรีนี้ได้ง่าย ๆ เพียงแค่ผู้เล่นทุกท่าน สมัครสล็อต เข้าใช้บริการที่ เว็บสล็อต888เว็บตรง ของเรา ก็สามารถเข้ามารับโปรโมชั่น สล็อตแตกหนัก นี้ได้เลยทันที คุณสามารถ สมัครสล็อตเครดิตฟรี เข้ามาทำการเดิมพันได้ทุกตัว เกมสล็อต โดยที่ไม่ต้องทำการฝากเงินเข้ามาก่อน ซึ่งเงื่อนไขของโปรโมชั่นและการจากเครดิตฟรีนี้คือผู้เล่นทุกท่านจะต้องทำยอดเทิร์นตามที่เรากำหนดให้ได้ก่อน คุณถึงจะสามารถถอนเงินรางวัลออกไปได้
การทดลองเล่นสล็อตฟรี ผู้เล่นสามารถเล่นได้โดยไม่ต้องสมัครสมาชิก ซึ่งระบบทดลองเล่นถือว่าเป็นทางเลือกที่ดีสำหรับผู้เล่นที่ต้องการสนุกสนานและฝึกทักษะโดยไม่ต้องเสียค่าใช้จ่าย คุณจะได้ฝึกทักษะและเรียนรู้เกมไปพร้อม ๆ กัน คุณสามารถทดลองเล่นเกมต่าง ๆ และทำความเข้าใจกับกฎของเกมได้ก่อนที่จะลงเดิมพันด้วยเงินจริง สล็อตทดลองเล่นของเราเล่นฟรีทุกค่าย รองรับทุกระบบไม่ว่าจะเป็น iOS หรือ Android เล่นได้ทุกเกมแบบโดยไม่ต้องสมัครก่อน เล่นผ่านเว็บไซต์ง่าย ๆ ไม่ต้องทำการดาวน์โหลด เปิดประสบการณ์ใหม่ไปกับระบบทดลองเล่น เกมสล็อต ออนไลน์ที่ไม่เหมือนใคร นอกจากนี้คุณยังสามารถซื้อฟรีสปินฟรีจากระบบทดลองเล่นได้อีกด้วย ระบบลื่นไหล เล่นง่าย ไม่มีสะดุดแน่นอน
บริการ เกมสล็อต ออนไลน์จาก เว็บสล็อตใหม่ล่าสุด ที่มีลิขสิทธิ์แท้ 100% และเล่นได้โดยไม่ต้องไม่ผ่านเอเย่นต์ ซึ่งที่ เว็บสล็อตใหม่ล่าสุด คุณจะได้พบกับประสบการณ์การเล่นสล็อตที่ยอดเยี่ยมและปลอดภัยที่สุด ซึ่งมีตัว เกมสล็อต ออนไลน์ที่เป็นที่นิยมในปี 2024 โดยเราคัดสรรมาเพื่อคุณผู้เล่นทุกท่าน เกมสล็อต เว็บเราเป็นลิขสิทธิ์แท้ทั้งหมด โดยได้รับการจดทะเบียนอย่างถูกต้อง การเล่นและลงเดิมพันมีอัตราความปลอดภัยและสะดวกสบายสูง มีอัตราความสำเร็จและทำกำไรได้จำนวนมาก หากคุณได้เล่นกับ เว็บสล็อตใหม่ล่าสุด ที่มีลิขสิทธ์แท้ ไม่ผ่านเอเย่นต์ อัตราชนะก็จะเพิ่มขึ้นรัว ๆ การทำเงินจาก เกมสล็อต ทุกประเภทใน เว็บสล็อตใหม่ล่าสุด ของเรานั้นมีขั้นตอนที่เรียบง่ายไม่ซับซ้อน
เว็บสล็อตแท้ ต้องเล่นทุกวันได้อย่างอิสระ เราคัดเกม สล็อตแตกง่าย ที่ได้รับความนิยมเป้นอย่างมากในปี 2024 มาให้คุณ และเป็น เกมสล็อตออนไลน์ ที่สามารถฝากถอนผ่านระบบการเล่น สล็อตวอเลท ได้แม้จะไม่มีบัญชีธนาคาร การเข้ามาเล่น เกมสล็อต ออนไลน์กับนั้นเรารองรับการฝากถอนออโต้ทุกระบบธนาคาร การเงินสเถียรและจ่ายเงินเต็ม แถมทุกครั้งที่คุณเล่นแต่ละครั้งมีรางวัลเพิ่มขึ้นรัว ๆ อีกด้วย ฝากถอนง่ายไม่ติดขัดด้วยบริการ สล็อตวอเลท ที่มีมาตรฐานและคุณภาพสูง ซึ่ง สล็อตวอเลท มาพร้อมกับระบบความปลอดภัยด้านการเงินและระบบบริการที่ตอบโจทย์ผู้เล่นมากที่สุด ระบบ สล็อตวอเลท ของเรารองรับทุกแพลตฟอร์มและทุกช่องทาง สามารถทำรายการได้อย่างรวดเร็วทันใจ ซึ่งรับประกันว่าระบบ สล็อตวอเลท จะสร้างความประทับใจให้แก่ผู้เล่นทุกท่าน
สล็อต 888 เว็บตรง ไม่ผ่านเอเย่นต์ ไม่มี ขั้นต่ำ นั้นเป็นแพลตฟอร์มของ เกมสล็อตออนไลน์ ที่กำลังมาแรงและได้รับความนิยมมากในช่วงเวลานี้ ด้วยระบบที่มีความสะดวกสบายในการเข้าเล่นและมีความโปร่งใสที่สุดเป็นเพราะว่า สล็อต 888 เว็บตรง ไม่ผ่านเอเย่นต์ ไม่มี ขั้นต่ำ สามารถติดต่อโดยตรงกับเว็บแท้ 100% รับประกันว่าจะทำให้ผู้เล่นมั่นใจได้ว่าผู้เล่นทุกท่านั้นจะได้รับประสบการณ์การเล่นที่ยุติธรรมและปลอดภัยอย่างแน่นอน ซึ่งหนึ่งในจุดเด่นที่น่าสนใจของ สล็อต 888 เว็บตรง ไม่ผ่านเอเย่นต์ ไม่มี ขั้นต่ำ นั้นก็คือระบบการฝากถอนเงินไม่มีขั้นต่ำมอบให้สำหรับผู้เล่นทุกท่าน ให้ผู้เล่นสามารถฝากเงินเพื่อเริ่มเล่นเกมได้ทันที ไม่ว่าผู้เล่นจะมีงบประมาณมากหรือน้อยเพียงใดผู้เล่นทุกท่านก็สามารถเข้าร่วมสนุกกับ สล็อต 888 เว็บตรง ไม่ผ่านเอเย่นต์ ไม่มี ขั้นต่ำ ได้อย่างไม่มีข้อจำกัด ไม่เพียงเท่านั้นรูปแบบของการถอนเงินก็ทำได้อย่างง่ายดายและรวดเร็วทันใจ ทั้งหมดนี้ก็เพื่อให้ผู้เล่นสามารถเพลิดเพลินกับการเล่นสล็อตออนไลน์ให้ชนะเงินรางวัลแบบปัง ๆ ได้อย่างสะดวกสบายไม่ติดขัด
สล็อตเว็บตรง 888 ทางเข้า เล่นสล็อตที่รวดเร็วและสะดวกสบาย รองรับการลงเดิมพันสำหรับสมาชิกและนักเดิมพันทุกคนฟรีตลอด 24 ชั่วโมง เข้าเล่นง่าย ระบบเร็วทันใจไม่มีสะดุด แถมเรายังมีการอัพเดท สล็อตเว็บตรง 888 ทางเข้า เล่น เกมสล็อตออนไลน์ ปั่นสล็อตทุกสัปดาห์เพื่อป้องกันมิจฉาชีพที่อาจจะแอบอ้างและอาจจะทำให้คุณเสียทรัพย์สิน เราคำนึงถึงความปลอดภัยในการเข้าเล่นของสมาชิกทุกท่านมาเป็นอันดับแรก เพียงแค่สมัครสมาชิกก็สามารถลงทะเบียนผ่านทาง สล็อตเว็บตรง 888 ทางเข้า เว็บของเราได้ทันที เปิดใจเข้าเล่นกับเราวันนี้ เล่นฟรีไม่มีจำกัด
เราคือ เว็บตรงapiแท้ ให้บริการเกม สล็อตแตกง่าย ที่สามารถเชื่อมต่อกับ เว็บสล็อตใหม่ล่าสุด ได้โดยตรง ทำให้ระบบที่ผู้เล่นจะลงเดิมพันมีความยุติธรรมสูง ลงทุนง่ายกว่าและปลอดภัยหายห่วง คุณสามารถเริ่มต้นเดิมพันและ ปั่นสล็อต ได้ง่าย เล่นกับเจ้าของค่าย สล็อต888 ได้โดยตรงไม่มีการโกงเกิดขึ้น 100% ยิ่งเล่นมาก ยิ่งหาเงินได้มาก API คือเส้นทางใหม่ที่พร้อมให้บริการ สล็อต888เว็บตรง ตามความต้องการนักเดิมพันในช่องทางที่ง่ายกว่า เราเป็นตัวจริงเรื่องการเดิมพันเพราะ เว็บตรงapiแท้ ของเราส่งตรงระบบ API มาจากต่างประเทศ
ใครที่ไม่เคยลงทุน ปั่นสล็อต กันมาก่อนคุณไม่ต้องกังวลอีกต่อไป เพราะ เว็บสล็อตใหม่ล่าสุด ของเราพร้อมจะพาทุกท่านเข้ามาพบช่องทาง สล็อตแจกจริง พารวย แน่นอนว่าสามารถสร้างเงินกันได้ง่ายมากที่สุด เกมสล็อต จาก สล็อต888เว็บตรง ของเรา คือเกมพิเศษที่สามารถสร้างเงินได้ไวกอบโกยกำไรได้มาก ซึ่งตัวเกม สล็อตแจกจริง สามารถทำความเข้าใจได้ง่าย เพียงแค่กดปุ่มเพื่อให้หมุนวงล้อเท่านั้น ท่านก็สามารถคว้าโบนัสและแจ็คพอตแตกหนักของตัวเกมได้เลยทันที ทวีคูณเงินรางวัลของทุกท่าน ไม่มีการกำหนดขั้นต่ำในการลงทุน สล็อตแตกง่าย อีกด้วย ถือได้ว่าพบช่องทางใหม่ที่สามารถสร้างรายได้เสริมได้ทุกวันอีกด้วย
เทคนิคในการเล่น เกมสล็อต ออนไลน์ให้ชนะหรือ สูตรสล็อต นั้นเป็นเทคนิคหรือวิธีการที่ผู้เล่นหรือนักเดิมพันสล็อตออนไลน์ส่วนใหญ่ใช้เพื่อเพิ่มโอกาสในการเล่นให้ชนะ เกมสล็อต ออนไลน์ แม้ว่า เกมสล็อต ออนไลน์นั้นจะเป็นเกมออนไลน์ที่มักจะต้องพึ่งพาโชคลาภเป็นหลัก แต่ก็ยังมีบางกลยุทธ์บางเทคนิคหรือที่เรียกกันว่า สูตรสล็อต โดยส่วนใหญ่มาจาก สล็อต เว็บตรงไม่ผ่านเอเย่นต์ไม่มีขั้นต่ำ ของเราซึ่ง สูตรสล็อต เหล่านี้จะสามารถช่วยให้ผู้เล่นหรือนักเดิมพัน เกมสล็อต ออนไลน์นั้นทำเพื่อเพิ่มโอกาสในการชนะซึ่งสูตรในการเล่น เกมสล็อต ออนไลน์ให้ชนะนั้นก็จะมีแตกต่างกันไปในแต่ละตัว เกมสล็อต ออนไลน์ โดยเว็บไซต์ www.reproduction-online.org ของเราก็ได้รวบรวมเทคนิคหรือ สูตรสล็อตแตกง่าย จากค่ายต่าง ๆ มี สูตรสล็อต ครบเกือบทุก เกมสล็อต ออนไลน์
ก่อนที่ผู้เล่นทุกท่านจะเริ่มเล่นเกม สล็อตแตกหนัก ผู้เล่นทุกท่านควรศึกษาข้อมูลของ เกมสล็อตออนไลน์ ที่คุณสนใจก่อนโดยพิจารณาในองค์ประกอบต่าง ๆ เช่น อัตราการจ่ายเงิน (RTP), ความผันผวนของเกม และฟีเจอร์พิเศษต่างๆ เว็บไซต์ เกมสล็อตออนไลน์ ของเราได้ให้ความรู้ไว้ ซึ่งเป็นแหล่งข้อมูลที่ดีในการหาเกม สล็อตแตกหนัก ที่มีการจ่ายรางวัลสูงและแตกบ่อยแตกหนัก ซึ่งการเลือกเล่น เกมสล็อต กับเว็บไซต์ www.reproduction-online.org ของเรานั้นจะทำให้ผู้เล่นทุกท่านมั่นใจได้ว่าการเล่นเกม สล็อตแตกหนัก ของท่านจะไม่พบอุปสรรคใด ๆ เพราะเกม สล็อตแตกหนัก ของเว็บไซต์เรานั้นใช้ซอฟต์แวร์จากผู้พัฒนาชั้นนำ เช่น NetEnt, Microgaming หรือ Playtech ซึ่งมีชื่อเสียงในการผลิต เกมสล็อตออนไลน์ คุณภาพจำนวนมาก เช่น เกมสล็อตออนไลน์ Mega Moolah หนึ่งในเกม สล็อตแตกหนัก ที่มีชื่อเสียงมากที่สุดในด้านการจ่ายแจ็คพอต เพราะแตกง่ายมากและมักจะจ่ายเงินรางวัลใหญ่อยู่เสมอ หรือ เกมสล็อตออนไลน์ Book of Ra เกม สล็อตแตกหนัก ที่มาในรูปแบบธีมอียิปต์ที่มีฟีเจอร์ฟรีสปินเกิดขึ้นบ่อยครั้งและมีสัญลักษณ์พิเศษที่จะคอยช่วยเพิ่มโอกาสในการชนะรางวัลของผู้เล่น หรือ เกมสล็อตออนไลน์ Starburst เป็นเกม สล็อตแตกหนัก ที่มีการจ่ายเงินรางวัลสูงเช่นกัน ถึงแม้จะไม่มีแจ็คพอตใหญ่บ่อยครั้ง แต่เป็นเกม สล็อตแตกหนัก ที่มีการชนะในช่วงรางวัลเล็ก ๆ บ่อยมาก ๆ และมีผู้เล่นหลายท่านชนะเงินรางวัลเล็ก ๆ เก็บจนเป็นเงินรางวัลก้อนใหญ่ได้ เป็นต้น
คำถามที่พบบ่อยเกี่ยวกับเกมสล็อตออนไลน์
สำหรับนักเดิมพันท่านใดที่มีข้อสงสัยเกี่ยวกับการใช้งานภายใน เว็บสล็อตออนไลน์ นั้น ทางเว็บไซต์ www.reproduction-online.org ของเราจึงได้ทำการรวบรวมคำถามที่พบบ่อยที่สุด พร้อมตอบคำถามอย่างชัดเจน เพื่อช่วยให้ผู้เล่นสามารถใช้งานในเว็บ สล็อตออนไลน์ ได้ง่ายมากยิ่งขึ้น ซึ่งคำถามที่พบบ่อยใน เว็บสล็อต ของเรา มีดังนี้
สำหรับการสมัครสมาชิกในเว็บ สล็อตออนไลน์ ของเรานั้นไม่จำเป็นต้องเตรียมเอกสารอะไรเลย ขอเพียงแค่ผู้เล่นมีความพร้อมที่จะเดิมพัน สล็อต ออนไลน์ก็สามารถกดสมัครสมาชิกกับเว็บไซต์ของเราได้เลยทันที
สำหรับการสมัครสมาชิกด้วยระบบ สล็อต Auto ในเว็บ สล็อต ของเรานั้น มีความง่ายจริง ทำให้ผู้เล่นสามารถสมัครสมาชิกด้วยตัวเองได้อย่างง่ายดาย โดยที่ไม่ต้องเสียเวลาไปกับการสมัครผ่าน แอพพลิเคชั่น Line หรือเอเย่นต์อีกต่อไป
สำหรับการฝากถอนในเว็บ สล็อต888 ของเรานั้นไม่จำเป็นต้องมีบัญชีธนาคารคือเรื่องจริง เพราะเว็บไซต์ของเรามีระบบฝากถอนที่ใช้งานผ่าน สล็อต Wallet หรือกระเป๋าเงินดิจิตอล ผู้เล่นไม่จำเป็นต้องมีบัญชีธนาคารก็สามารถฝากถอนกับทางเราได้
สำหรับการฝากถอนในเว็บ สล็อตออนไลน์ ของเรานั้นไม่มีกำหนดขั้นต่ำในการทำรายการ ผู้เล่นที่มีความกังวลกับเรื่องนี้อยู่นั้น เกิดความสบายใจได้เลย ถึงแม้ว่าผู้เล่นจะมีเงินทุนน้อยก็สามารถทำรายการฝากเงินกับเราได้ และถอนเงินได้ตามต้องการอีกด้วย
สำหรับการเข้าใช้งานโหมด ทดลองเล่นสล็อตฟรี นั้น ผู้เล่นสามารถเข้าใช้งานได้อย่างง่ายดาย โดยเข้ามาที่หน้าเว็บไซต์ของเราและเลือกตรงที่แถบเมนู “ ทดลองเล่นสล็อตฟรี ” จากนั้นระบบของเราจะพาท่านเข้าสู่หน้าทดลองเล่น สล็อต ฟรีทันที และในการใช้งานโหมด ทดลองเล่น นั้น ผู้เล่นไม่จำเป็นต้องสมัครสมาชิกก็สามารถเล่นได้อีกด้วย
การใช้งานโหมด ทดลองเล่นสล็อต นั้นผู้เล่นไม่ต้องเติมเงินใด ๆ ทั้งสิ้น เพราะ สมัครสล็อตเครดิตฟรี กับเว็บไซต์ www.reproduction-online.org ของเรา จะมีเครดิตฟรีให้กับคุณ ซึ่งคุณสามารถนำเครดิตฟรีไปใช้เพื่อ ทดลองเล่นสล็อต ได้เลยทันที และเมื่อเครดิตฟรีหมดก็สามารถขอใหม่ได้ แต่เครดิตฟรีที่ได้รับจากการ สมัครสล็อตเครดิตฟรี ที่เว็บไซต์ของเรามอบให้นั้น เป็นเครดิตฟรีสำหรับการ ทดลองเล่นสล็อต เท่านั้น ไม่สามารถถอนเป็นเงินสดได้
สำหรับการกดรับโปรโมชั่น สล็อต888 คือการที่เว็บ สล็อตแจกจริง ให้กับผู้เล่นทุกท่านในส่วนของ โปรโมชั่นสล็อต โดยผู้เล่นสามารถกดรับผ่านหน้าเว็บไซต์ www.reproduction-online.org ของเราได้เลย แต่ผู้เล่นจะต้องทำตามเงื่อนไขและข้อตกลงของ โปรโมชั่นสล็อต ที่คุณเลือกให้ถูกต้องและครบถ้วน เพียงเท่านี้คุณก็สามารถแจ้งรับ โปรโมชั่นสล็อต ผ่านหน้าเว็บไซต์ www.reproduction-online.org ของเราได้เลยทันที
โปรโมชั่นสล็อต ในเว็บไซต์ www.reproduction-online.org ของเรานั้นมีระยะวันและเวลาที่กำหนดไว้ ผู้เล่นจะต้องอ่านรายละเอียดของโปรโมชั่นนั้น ๆ ให้ครบถ้วน เพื่อป้องกันการสูญเสียสิทธิ์การรับ โปรโมชั่นสล็อต ของท่าน
หากผู้เล่นพบเจอกับปัญหาในการใช้งานภายในเว็บไซต์ของเรานั้น คุณสามารถติดต่อมาที่เว็บไซต์ www.reproduction-online.org ของเราโดยตรงได้เลย ทางเว็บไซต์ www.reproduction-online.org ของเราจะมีระบบบริการ สมาชิกสล็อต ตลอด 24 ชั่วโมง อีกทั้งยังมีทีมงานฝ่ายสนับสนุนผู้เล่น คอยให้คำแนะนำผู้เล่นทุกท่านด้วยคนจริง ๆ ผ่านการอบรมและฝึกฝนมาเป็นอย่างดี ไม่ใช่ระบบ Ai และทางเราจะเร่งดำเนินการแก้ไขปัญหาต่าง ๆ ให้กับผู้เล่นทันทีเพื่อสร้างความประทับใจให้แก่ผู้เล่นทุกท่าน ให้ผู้เล่นทุกท่านรู้สึกถึงความอบอุ่นและมั่นคงปลอดภัยตลอดระยะเวลาในการเข้าเล่นเกมสล็อตออนไลน์
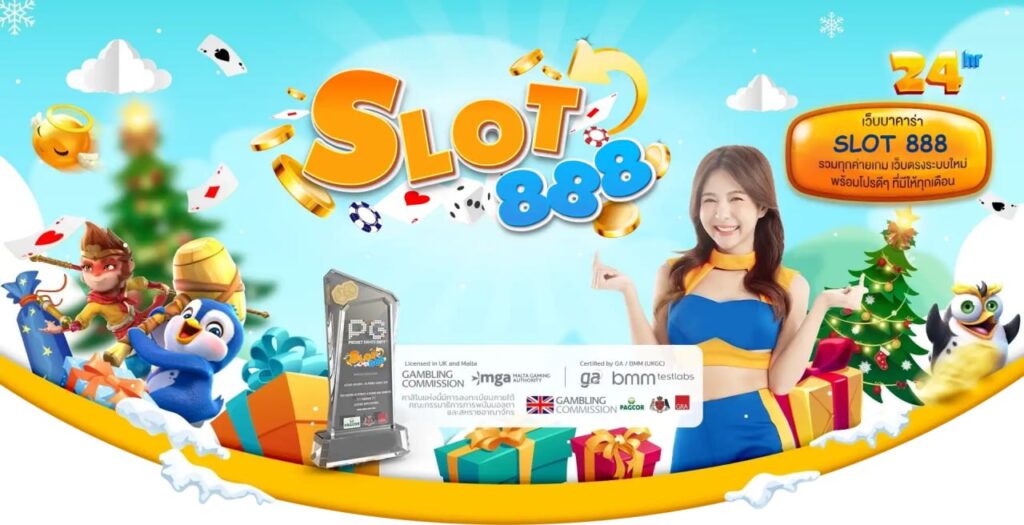

An official website of the United States government
The .gov means it’s official. Federal government websites often end in .gov or .mil. Before sharing sensitive information, make sure you’re on a federal government site.
The site is secure. The https:// ensures that you are connecting to the official website and that any information you provide is encrypted and transmitted securely.
- Publications
- Account settings
Preview improvements coming to the PMC website in October 2024. Learn More or Try it out now .
- Advanced Search
- Journal List
- HHS Author Manuscripts

The Epic Journey of Sperm Through the Female Reproductive Tract
Millions or billions of sperm are deposited by artificial insemination or natural mating into the cow reproductive tract but only a few arrive at the site of fertilization and only one fertilizes an oocyte. The remarkable journey that successful sperm take to reach an oocyte is long and tortuous, and includes movement through viscous fluid, avoiding dead ends and hostile immune cells. The privileged collection of sperm that complete this journey must pass selection steps in the vagina, cervix, uterus, utero-tubal junction, and oviduct. In many locations in the female reproductive tract, sperm interact with the epithelium and the luminal fluid, which can affect sperm motility and function. Sperm must also be tolerated by the immune system of the female for an adequate time to allow fertilization to occur. This review emphasizes literature about cattle but also includes work in other species that emphasizes critical broad concepts. Although all parts of the female reproductive tract are reviewed, particular attention is given to the sperm destination, the oviduct.
Introduction
Normally only one sperm fertilizes an oocyte despite that billions of sperm are deposited by natural mating into the vagina, or millions are deposited by artificial insemination into the uterus of a cow. The remarkable journey that successful sperm take to reach the oocyte is long and tortuous, filled with viscous fluid, dead ends, and potentially hostile immune cells. Rather than a simple race to get to the oocyte, there is much evidence that complex mechanisms influence sperm transport, immunological tolerance of sperm, sperm selection, sperm storage and release, all before actual fertilization. At steps along the way to the site of fertilization, sperm may interact with the fluid in which they are suspended and the epithelium lining the tract. The very dynamic process of sperm transport helps ensure that there is an appropriate number of fertile sperm at the site of fertilization so that the oocyte can be fertilized by only one sperm. This review considers sperm interaction with fluid in the reproductive tract as well as sperm adhesion to the epithelium. It also reviews how sperm, foreign cells in the female reproductive tract, are tolerated by the immune system. Although it emphasizes literature about cattle, concepts developed in other species are included.
Sperm in the Vagina and Cervix
Sperm are transported through the vagina, cervix, and uterus to the oviduct where they can fertilize oocytes. In cattle and many other mammals, estrus occurs before ovulation so sperm are deposited in the female reproductive tract before ovulation. At normal copulation in cattle, semen is deposited in the cranial vagina. Vaginal fluid is the first luminal medium to which sperm are exposed after semen deposition. The acidic pH of the vagina makes it inhospitable for sperm, although buffers found in semen neutralize the local pH. The cow produces a large volume of vaginal fluid and up to 100 ml can accumulate (reviewed by ( Rutllant et al. , 2005 ). The rheological properties of vaginal fluid appear to influence sperm motility characteristics, although fertilizing sperm may spend only a short time in the vagina ( Rutllant et al. , 2005 ).
It is likely that bovine sperm, like human sperm ( Suarez and Pacey, 2006 ), that are candidates to fertilize oocytes enter the cervical canal quickly avoiding damage due to the low vaginal pH. The cervix contains many folds and grooves that are filled with mucus. The mucus within the canal is a major barrier to sperm, particularly those that have abnormal motility ( Katz et al. , 1997 ). The composition and structure of cervical mucus changes near estrus, allowing sperm with normal motility to advance, typically through what have been called “privileged paths” that are found in the grooves produced by folds that extend through the cervical canal ( Mullins and Saacke, 1989 ). A microfluidic model has confirmed that sperm migration through these privileged paths is controlled by microgrooves and a gentle flow of fluid ( Tung et al. , 2015b ).
Sperm are foreign cells and can induce an immune response in the cervix. In rabbits, neutrophil infiltration was observed within 30 min of mating ( Tyler, 1977 ). Immunoglobulins IgG and IgA ( Kutteh et al. , 1996 ) and complement proteins have been detected in human cervical mucus ( Mathur et al. , 1988 ). Therefore, sperm retained in the cervix might be attacked by the immune system before moving into the uterus.
Sperm in the Uterus
After natural mating, sperm move from the cervical canal into the uterus. In cattle, artificial insemination (AI) is used frequently. When performing AI, the technician deposits semen directly into the uterine body, so sperm do not enter the vagina and cervix. Depositing sperm directly in the uterus reduces the number of sperm needed for routine AI to 10–20 million ( Moore and Hasler, 2017 ). As few as 2 million sperm are often inseminated when using sperm separated based on their sex chromosome, a process used to bias the sex of offspring ( DeJarnette et al. , 2009 ). Experiments in which the uterotubal junction (UTJ) in heifers was ligated at various times after mating revealed that it took 6–8 hr for sperm to move through the cervix and uterus to infiltrate the oviduct in numbers sufficient for oocyte fertilization ( Wilmut and Hunter, 1984 ). Sperm are transported through the uterus with the aid of uterine smooth muscle contractions in the direction of the oviduct ( Hawk, 1987 ). To measure fluid movement and uterine contractions, technetium-labeled albumin-macrospheres were deposited in the uterus of women. These macrospheres (5–40 μm diameter) could be detected by high-resolution ultrasound. They were transported from the uterus to the oviduct more rapidly in the late follicular phase ( Kunz et al. , 1996 ) which, along with other experiments, indicates that uterine contractions that transport sperm are under endocrine control. Further, this result demonstrates that materials in addition to sperm can move through the UTJ.
Sperm in the uterus of cattle and other species are retained in uterine glands in low numbers per gland ( Hunter, 1995 , Rijsselaere et al. , 2004 ). Retention, at least in swine, is accomplished by sperm binding to uterine epithelial cells ( Rath et al. , 2016 ). Sperm attachment to uterine cells stimulates the production of both pro- and anti-inflammatory cytokines ( Lovell and Getty, 1968 ). There is evidence that porcine sperm bind to sialic acid-containing glycans on the surface of uterine epithelial cells ( Rath et al. , 2016 ). For example, a sialic acid lectin that recognizes sialic acid binds to uterine epithelial cells and blocks sperm binding, in vitro . Although it is not clear whether many sperm in uterine glands move into the oviduct, the fate of the majority of sperm in the uterus is elimination.
Rapid removal of sperm may help reduce the acquired immune response against sperm ( Hansen, 2011 ). Little is known about the immune response elicited by semen deposition in cattle but it has been studied more in rodents and horses ( Katila, 2012 , Bromfield, 2014 , Christoffersen and Troedsson, 2017 ). The primary function of the inflammatory response is to clear excess sperm, seminal debris and bacteria from the uterus. Following semen deposition, there is an infiltration of polymorphonuclear leukocytes. In addition to activation of innate immunity, adaptive immunity is also involved. Several classes of antibodies have been isolated from uterine fluid. In addition to cytokines released from the uterine endometrium, seminal plasma itself contains immune system modulators that affect uterine and oviduct immune cells ( Robertson, 2007 , Schjenken and Robertson, 2014 and 2015 ). There is evidence that a seminal vesicle protein may allow the uterus to tolerate sperm ( Kawano et al. , 2014 ). Interestingly, the seminal fluid fraction of semen also improves preimplantation development and has interesting long-term effects on offspring ( Bromfield et al. , 2014 ). This non-traditional role of seminal plasma has been studied most in rodents; the amount of seminal plasma in cattle that mate normally is low and even lower when artificial insemination is used.
Sperm Entry into the Oviduct through the Utero-tubal Junction
In the bovine UTJ, sperm move through a slit-like lumen with a mucosal pad and into the lower portion of the oviduct, the isthmus, which contains 4–8 primary grooves in tubal segment ( Wrobel et al. , 1993 ). Compared to the major part of the upper oviduct, the ampulla, the isthmus has a narrower lumen with fewer folds but a thicker layer of smooth muscle. Although macrospheres seem to have the ability to pass through the UTJ (discussed above), there is evidence that sperm, at least in mice, require a specific protein to be recognized and to pass through the UTJ into the isthmus. Mouse sperm deficient in ADAM3, due to mutation of the ADAM3 gene or genes whose products affect ADAM3 are not detected beyond the UTJ ( Nakanishi et al. , 2004 , Yamaguchi et al. , 2006 , Yamaguchi et al. , 2009 , Okabe, 2013 ). Even if sperm from a chimeric male derived from a normal and a mutant embryo were deposited, only the normal sperm moved into the oviduct ( Nakanishi et al. , 2004 ). Thus, the presence of normal sperm does not aid in opening the UTJ to allow ADAM3 mutant sperm to pass into the oviduct.
In addition to ADAM3, there also appears to be a rheological barrier in the porcine UTJ, perhaps the viscous mucus present in the grooves of this structure ( Hunter, 2002 , Tienthai, 2015 ). The rabbit and mouse UTJ and oviduct fluid contain proteoglycans with sulfated glycosaminoglycan chains and hyaluronan ( Jansen, 1978 , Suarez et al. , 1997 ). In addition to changing the viscosity and affecting sperm motility, the abundance of hyaluronan in fluid and its receptor, CD44 on the epithelial cells of the UTJ, suggest that CD44 signal transduction might affect the function of the UTJ and lower oviduct ( Bergqvist et al. , 2005a , Bergqvist et al. , 2005b ).
In cattle and other species, there appears to be a valve at the UTJ that can constrict the lumen, restricting sperm entry. This valve is formed by a vascular plexus and surrounded by a thick muscle layer that, in total, can contract the lumen ( Wrobel et al. , 1993 ). The physical constriction, mucus barrier and protein signature requirements emphasize how stringently entrance to the oviduct is regulated.
Sperm in the Oviduct
Once sperm enter the lower oviduct, the isthmus, they can bind to the epithelial cell surface or remain in oviduct fluid. Many studies of the intact oviduct have been performed in mice because the uterus and oviduct can be transilluminated so that sperm can be observed ( Demott and Suarez, 1992 ). Sperm from transgenic mice that have enhanced green fluorescent protein in their acrosomes and red fluorescent protein in their midpiece mitochondria have been followed in the female tract after natural mating ( La Spina et al. , 2016 ). The location of live sperm and their acrosomal status can be followed using fluorescence microscopy.
When sperm in the lumen of the isthmus were observed, groups of sperm were carried by fluid that was moved alternately toward the uterus and then toward the ampulla (back and forth) by contractions of oviduct smooth muscle ( Ishikawa et al. , 2016 ). These contractions were not observed in the ampulla. Most of the sperm in the isthmus were acrosome-intact ( La Spina et al. , 2016 ). Relatively few sperm were found in the ampulla and most were acrosome-reacted ( La Spina et al. , 2016 , Muro et al. , 2016 ), consistent with the recent evidence that the acrosome reaction of fertilizing mouse sperm occurs prior to contact with the cumulus-oocyte complex ( Jin et al. , 2011 , La Spina et al. , 2016 ).
Oviduct Fluid Affects Sperm Function
The fluid in the oviduct is highly viscous, unlike the culture medium in which studies of mammalian fertilization are usually performed. Fluid viscosity is often overlooked in studies of sperm function within the oviduct. More viscous fluid has more internal friction so the wake from a sperm swimming in viscous medium is relatively small compared to less viscous medium ( Kirkman-Brown and Smith, 2011 ). Studies of human sperm demonstrate that resistance of the fluid to be moved results in a sperm tail with multiple bends while beating ( Kirkman-Brown and Smith, 2011 , Hyakutake et al. , 2015 ). In contrast, in less viscous medium, the tail has fewer bends and, instead, remains mostly straight while simply swinging or flapping back and forth ( Kirkman-Brown and Smith, 2011 , Hyakutake et al. , 2015 ). Consequently, in viscous fluid, a motile sperm will have less side-to-side movement (yaw) than in a standard viscosity medium ( Kirkman-Brown and Smith, 2011 ). Sperm also tend to swim near and against solid surfaces, for example epithelial walls or the corners of microchannels ( Denissenko et al. , 2012 ). Sperm that are close to the channel wall swim faster than those moving in the center of the channel ( El-Sherry et al. , 2014 ). Viscoelastic medium induces bovine sperm to swim in coordinated groups that may facilitate sperm migration ( Tung et al. , 2017 ). The majority of sperm orient their swimming so that they swim against the flow of medium when the flow rate is intermediate (33–134 μm/sec) ( Miki and Clapham, 2013 , El-Sherry et al. , 2014 , Tung et al. , 2015a ). This appears to guide sperm upstream in oviduct fluid ( Miki and Clapham, 2013 ). There is controversy about whether a signaling process in sperm aids in orienting sperm in the upstream direction or if sperm rheotaxis is a passive process ( Miki and Clapham, 2013 , Hyakutake et al. , 2015 ).
Interestingly, the viscosity of oviduct fluid varies during the estrous cycle; tenacious mucus is found in the rabbit oviduct lumen at estrus and disappears after ovulation ( Jansen, 1978 ). Most studies of sperm-oviduct interaction or fertilization have used standard culture medium and ignored its low viscosity, compared to oviduct fluid. A few have tried to recapitulate the viscosity of oviduct fluid by adding components like methylcellulose or polyvinylpyrrolidone to medium ( Suarez and Dai, 1992 , Alasmari et al. , 2013 , Gonzalez-Abreu et al. , 2017 ). In addition to effects on normal motility, discussed above, physiological viscosity converts the wild thrashing motion and high yaw of hyperactivated sperm to motility with less yaw and a more forward movement ( Suarez and Dai, 1992 ).
In addition to the rheological properties of oviduct fluid, specific components of oviduct fluid such as secreted proteins, proteoglycans, and lipids may influence fertilization by affecting sperm function ( Coy et al. , 2010 , Killian, 2011 ). This complex fluid can affect sperm prior to encountering the oocyte and during fertilization ( Rodriguez-Martinez, 2007 , Killian, 2011 ). For example, bovine sperm take up phospholipids that are abundant in oviduct fluid ( Killian et al. , 1989 )( Evans and Setchell, 1978 ). Oviduct fluid glutathione peroxidase, superoxide dismutase and catalase can protect bovine sperm from damage by reactive oxygen species that may otherwise reduce sperm viability and motility ( Lapointe and Bilodeau, 2003 ). Proteoglycans found in oviduct fluid promote capacitation of bovine sperm through their glycosaminoglycan side chains ( Parrish et al. , 1989 , Bergqvist et al. , 2006 ).
Oviduct fluid components, for example glycosaminoglycans, can also cause proteolysis or loss of sperm membrane proteins, including those that are implicated in sperm binding to the oviduct epithelium. The best studied of these proteins originate from accessory gland secretions and bind to sperm at ejaculation. Some bovine Binder of Sperm (BSPs) and porcine sperm adhesins are lost as sperm are capacitated ( Topfer-Petersen et al. , 2008 , Hung and Suarez, 2010 ). Although the significance of protein loss or proteolysis is uncertain, in sperm bound to the oviduct epithelium, it might contribute to their release prior to fertilization ( Topfer-Petersen et al. , 2008 , Hung and Suarez, 2010 ).
In addition to losing proteins, sperm also gain proteins while they reside in the oviduct. The first of two examples is Oviduct Specific Glycoprotein (OGP) or oviductin, also known as OVGP1, found in oviducts of many mammals. Although it has homology to the chitinase family of proteins, OGP does not have enzymatic activity ( Jaffe et al. , 1996 , Araki et al. , 2003 ). Bovine sperm incubated in OGP have improved motility and viability ( Abe et al. , 1995 ). Hamster sperm treated with recombinant OGP have increased phosphorylation of tyrosine residues on proteins, an indication that capacitation was enhanced ( Yang et al. , 2015 ). There is also evidence in mice and swine that OGP binds to the zona pellucida to increase fertilization success by rendering the zona matrix more permissive to penetration by sperm ( Lyng and Shur, 2009 , Algarra et al. , 2016 ).
A second example of an oviduct protein that affects sperm is osteopontin. Although it is already bound to bovine sperm before semen is deposited in females ( Erikson et al. , 2007 ), addition of osteopontin during in vitro fertilization reduces polyspermy ( Goncalves et al. , 2008 ). Neither osteopontin nor OGP is necessary for fertility in mice because animals deficient in each are fertile ( Rittling et al. , 1998 , Araki et al. , 2003 ).
In addition to oviduct fluid proteins being added as peripheral membrane proteins, integral membrane proteins could be added by fusion with sperm of oviductosomes secreted by the oviduct. For example, a portion of the major Ca 2+ efflux pump is added to mouse sperm by oviduct exosomes ( Al-Dossary et al. , 2015 ). The proteins secreted by bovine oviduct cells and found in oviduct fluid have recently been profiled and include growth factors, metabolic regulators, immune modulators, enzymes and extracellular matrix components ( Lamy et al. , 2016 , Pillai et al. , 2017 ). They function in immune homeostasis, gamete maturation, fertilization and early development ( Pillai et al. , 2017 ). The abundance of some depend on the stage of the estrous cycle and whether they were found in oviducts ipsilateral or contralateral to the ovary that ovulated ( Lamy et al. , 2016 ).
The Oviduct as a Functional Sperm Reservoir
The oviduct, along with the UTJ in some species, appears to be the major location in which sperm are stored before fertilization. In contrast, although sperm are retained in the cervix or uterus, it is not clear that they are eventually released to move to the oviduct. So the UTJ and oviduct appear to be the major sperm storage sites in many mammals. To be a true “functional sperm reservoir”, as coined by Hunter, ( Hunter et al. , 1980 ), in addition to retaining sperm, the oviduct must affect sperm function and lengthen sperm lifespan beyond the inherent longevity of sperm ( Orr and Zuk, 2014 ). More than simple adhesion occurs because binding to the oviduct epithelium prolongs the lifespan of sperm and suppresses capacitation and motility ( Pollard et al. , 1991 , Rodriguez-Martinez, 2007 , Hung and Suarez, 2010 )( Rodriguez-Martinez et al. , 2005 ). Thus, the oviduct isthmus meets these requirements. But the ability of sperm reservoirs described in a variety of species to prolong the lifespan of a highly differentiated and transcriptionally inactive cell is enigmatic.
The reservoir also releases a finite number of stored sperm, acting as a buffer for sperm number to prevent polyspermy but still provide an appropriate number of fertile sperm to the upper oviduct ( Hunter and Leglise, 1971b ). The isthmic epithelium binds and retains preferentially sperm that have intact acrosomes and normal morphology ( Teijeiro and Marini, 2012 )( Teijeiro et al. , 2011 ). All together, the isthmus functions to increase the probability that a suitable number of fertile sperm are present at the site of fertilization.
The Oviduct Epithelium Retains Sperm and Modulates Sperm Function
In mammals, the oviduct epithelium binds and retains sperm so they accumulate to form the reservoir. Adhesion is very specific. The sperm head binds to oviduct epithelial cells but not all cells ( Pacey et al. , 1995 , Kervancioglu et al. , 2000 ). And the ability of sperm binding to maintain viability is not a common property of all cells ( Boilard et al. , 2002 ). The ability to maintain viability requires direct contact between sperm and oviduct epithelial cells ( Dobrinski et al. , 1997 , Murray and Smith, 1997 , Smith and Nothnick, 1997 ). Adhesion to the oviduct regulates sperm capacitation ( Dobrinski et al. , 1997 , Boilard et al. , 2002 , Fazeli et al. , 2003 ) and suppresses the normal increase in sperm intracellular free calcium that occurs during capacitation ( Dobrinski et al. , 1996 , Dobrinski et al. , 1997 ).
Studies performed in several mammals have concluded that glycans are the components in oviduct epithelial cells that bind sperm ( Lefebvre et al. , 1997 , Green et al. , 2001 , Suarez, 2001 , Cortes et al. , 2004 , Topfer-Petersen et al. , 2008 ). The evidence in most studies underpinning a role for oviduct glycans is a competition assay in which different glycans are added to sperm before challenging these sperm by allowing them to bind oviduct epithelial cells in vitro. If few sperm bind to oviduct cells, this result is interpreted as an indication that the specific glycan is related to the authentic oviduct glycan that binds sperm. A frequent problem with these studies is that most test high concentrations of a small number of monosaccharides or small oligosaccharides.
Identification of Glycans that Bind Porcine Sperm Using a Glycan Array
The development of glycans immobilized on an array provided an opportunity to test hundreds of glycans for their ability to bind sperm. Using such an array, nearly 400 glycans were tested for their ability to bind porcine sperm ( Kadirvel et al. , 2012 ). All the glycans that bound sperm contained one of two glycan motifs, either a Lewis X trisaccharide (Le X ) or a structure with with core mannose and two antennae terminating in the sialylated lactosamine trisaccharide bi-SiaLN or in simply lactosamine ( Figure 1 ). There were several examples demonstrating that sperm bound these two motifs with high specificity. In all sialic acid-containing structures that bound sperm, sialic acid was linked to the 6 position of galactose; structures that were identical except that sialic acid was attached to galactose at the 3 position did not bind sperm. Furthermore, the branched structure on a mannose core was required because single sialylated lactosamine trisaccharides (Neu5Acα2–6Galβ1–4GlcNAc) did not bind sperm ( Kadirvel et al. , 2012 ).

Structures of glycans that bind bovine (Le A ) and porcine sperm (bi-SiaLN, bi-LN, and Le X ), and the related glycan that does not (LN). Le A is found on the bovine oviduct epithelium. bi-SiaLN is abundant on the epithelium of the porcine ampulla and isthmus including ciliated and non-ciliated cells. Le X is found in the porcine isthmus but not the ampulla.
The Le X trisaccharide was found as a monomer, dimer or trimer in the remaining glycans that bound sperm ( Kadirvel et al. , 2012 ). This trisaccharide is composed of Gal and Fuc linked to GlcNAc ( Figure 1 ). The Le X trisaccharide also bound sperm with high specificity; the closely related Lewis A trisaccharide (Le A , a positional isomer; the carbons in GlcNAc to which Gal and Fuc are linked are exchanged) did not bind porcine sperm. Contrarily, bovine sperm bind Le A but not Le X ( Suarez et al. , 1998 ). Binding specificity was further supported because porcine sperm did not bind to Galβ1–4GlcNAc; fucose substitution on Le X was necessary ( Kadirvel et al. , 2012 ).
To confirm that the glycans on the array that bound sperm were present in the oviduct isthmus and to determine the complete structures of the oviduct glycans that bound sperm, oviduct glycans and glycolipid structures were identified by tandem mass spectrometry ( Kadirvel et al. , 2012 ). The Le X and branched sialylated motifs (bi-SiaLN) that bound sperm were found on larger structures that were the most abundant of the complex-type glycans on epithelial cells ( Kadirvel et al. , 2012 ). Most of the complex-type oligosaccharides linked to proteins through asparagine residues were branched with two antennae and several had a sialyl residue on at least one terminus. Some biantennary glycans had both motifs, a sialyl residue on one terminus and a Lewis structure on the second. This kind of hybrid glycan was not present on the array but it is possible that, because it includes both motifs, it might bind sperm with highest affinity.
Because tandem mass spectrometry did not distinguish between Le A and Le X and between glycans with sialyl residues attached to the 6-carbon and the 3-carbon of Gal, an additional strategy was used. An antibody and specific lectin, Sambucus nigra agglutinin (SNA) were used that recognize sialic acid attached to galactose in an α-2,6 linkage preferentially and not sialic acid attached to galactose in an α-2,3 linkage ( Naito et al. , 2007 , Song et al. , 2011 ). Both reagents detected 6-sialylated structures that were abundant on the epithelium throughout the oviduct including on ciliated and non-ciliated cells ( Kadirvel et al. , 2012 ).
Similarly, an antibody to Le X was also used to confirm the identity of the oviduct Lewis trisaccharide structures identified by MS ( Kadirvel et al. , 2012 ). Interestingly, Le X was found in a punctate pattern at the luminal surface of porcine isthmic epithelial cells ( Machado et al. , 2014 ) but was not found in the ampulla.
bi-SiaLN and Le X Glycan Motifs Bind to the Porcine Sperm Head
The head is the portion of sperm that binds to the oviduct epithelium and is where ( Suarez et al. , 1991 ) authentic receptors for glycans with bi-SiaLN and/or Le X motifs should be localized. Fluorescein-labeled Le X and bi-SiaLN bound preferentially to the apical edge of the head in 60–70% sperm prior to capacitation ( Kadirvel et al. , 2012 , Machado et al. , 2014 ). Binding of fluoresceinated glycans could be displaced by an excess of the same glycan that did not have a fluorescent tag. The binding specificity was confirmed by testing sperm binding to oviduct glycans attached to Sepharose beads ( Figure 2 ). Tethering a motile cell to a solid phase glycan rather than a soluble glycan more closely mimics sperm binding to the oviduct and requires a higher affinity.

Sperm bind to oviduct cell aggregates isolated from the isthmus (A, porcine sperm) and beads to which Le A trisaccharide has been attached (B, bovine sperm).
Porcine Sperm Binding to Oviduct Cells Requires Glycans with bi-SiaLN and Le X
Experiments using immobilized glycans (i.e. the glycan array and glycans linked to Sepharose) showed that bi-SiaLN and Le X were each sufficient to tether a motile sperm. Necessity experiments were performed in which either the glycans or putative receptors were blocked. The result of blocking was assessed by sperm binding to aggregates of epithelial cells stripped from the isthmus ( Figure 2 ). Results of these experiments indicated that each glycan or glycan receptor was necessary for sperm to bind oviduct cells.
Receptors on Sperm for Oviduct Glycans
The identity of the sperm molecules that mediate binding to the oviduct is controversial. It appears that different species may use different adhesion molecules. Using bovine tissues, one group found that two oviduct proteins, the chaperones GRP78 and HSP60, bound to sperm ( Boilard et al. , 2004 ). In contrast, a second group, also using bovine sperm, proposed that oviduct plasma membrane annexins containing fucose bind to accessory gland proteins deposited on sperm at ejaculation ( Ignotz et al. , 2007 ). This result was a bit surprising because annexins are usually considered as cytosolic proteins and they lack signal peptides that would direct them through the secretory pathway to become fucosylated. A proteomic study found that annexin A1 is the most abundant protein in oviduct fluid ( Lamy et al. , 2016 ). Perhaps it is released into fluid without passing through the secretory pathway. But in the fluid, it would be expected to compete with annexin A1 located on oviduct epithelial cells and decrease sperm binding to the oviduct.
Studies of porcine sperm also implicated accessory gland secretions added to sperm ( Ekhlasi-Hundrieser et al. , 2005 , Topfer-Petersen et al. , 2008 ). The spermadhesin AQN1 originating from accessory gland secretions is a glycan-binding protein ( Ekhlasi-Hundrieser et al. , 2005 , Topfer-Petersen et al. , 2008 ). Spermadhesins represent 90% of the total boar seminal plasma protein and they become peripherally associated with the sperm plasma membrane after ejaculation ( Sanz et al. , 1993 ). Sperm AQN1 is reported to bind mannose and galactose residues on oviduct cells, but not Le X or bi-SiaLN structures ( Ekhlasi-Hundrieser et al. , 2005 ).
The observation that the accessory gland proteins do not bind Le X and bi-SiaLN motifs ( Ekhlasi-Hundrieser et al. , 2005 ) and sperm obtained from the cauda epididymis are still able to bind oviduct cells, although in reduced number ( Petrunkina et al. , 2001 ), suggested that other glycan receptors were important. Indeed, in cattle there is no evidence that the fertility of epididymal sperm, not exposed to accessory gland proteins, is lower that normal ejaculated semen that includes accessory gland secretions ( Amann and Griel, 1974 ). The fertility of cauda epididymal sperm motivated the investigation of glycan receptors on porcine sperm from the epididymis, which also avoided interference from the very abundant accessory gland proteins ( Silva et al. , 2014 ).
Membrane lysates from porcine cauda epididymal sperm were separated chromatographically and each fraction was subjected to SDS-PAGE, transferred to nitrocellulose and incubated with biotinylated Le X and bi-SiaLN. This “glycan blot” was used to identify proteins with appropriate glycan affinity. Several proteins were identified including the peripheral membrane protein MFG-E8, also known as lactadherin, P47 or SED1 ( Silva et al. , 2017 ). Competition experiments showed that lactadherin bound to oviduct cells and that inhibition reduced sperm binding ( Silva et al. , 2017 ).
Although there is compelling evidence that oviduct glycans are at least partially responsible for sperm binding, there is also evidence that sperm binding to oviduct epithelial cells is mediated to some degree by other interactions. Perturbation of glycans or their candidate receptors decreases sperm binding to oviduct cell aggregates by a maximum of 60% ( Kadirvel et al. , 2012 , Machado et al. , 2014 ). Protein-based interactions may account for the residual binding. For example, fibronectin from oviduct cells can bind α5β1 integrin on bovine sperm ( Osycka-Salut et al. , 2017 ) and the adhesion protein E-cadherin is found in both sperm and oviduct epithelial cells ( Caballero et al. , 2014 ). ( Pollard et al. , 1991 , Lefebvre et al. , 1995 )
Oviduct Epithelial Cells Respond to Sperm Binding
In addition to the effect of adhesion on sperm, sperm adhesion to the oviduct modifies the transcriptional profile of oviduct epithelial cells ( Fazeli et al. , 2004 , Georgiou et al. , 2005 , Georgiou et al. , 2007 , Lopez-Ubeda et al. , 2015 ). Genes related to the inflammatory response, molecular transport, protein trafficking, and cell-to-cell signaling are among those most affected by sperm ( Lopez-Ubeda et al. , 2015 ). In the sow, there is evidence that the ovary has a local effect on the transcriptome of the oviduct. Unilateral ovariectomy reduces expression of genes believed to be involved in sperm survival and early embryonic development ( Lopez-Ubeda et al. , 2016 ). The effect of sperm on the sperm reservoir appears conserved between birds and mammals. Infiltration of porcine sperm into the UTJ and rooster sperm into the chicken utero-vaginal junction alters the expression of genes involved in pH regulation and immune-modulation ( Atikuzzaman et al. , 2017 ). Even more surprisingly, the transcriptional response of oviduct cells is different in response to insemination of either X chromosome- or Y chromosome-bearing sperm ( Alminana et al. , 2014 ). Thus, the presence of sperm changes the behavior of oviduct cells in addition to its consequences for sperm. The result of altered production of specific proteins by oviduct cells is not clear.
Sperm Release from Oviduct Epithelial Cells
The fertilizing sperm must be released from the oviduct reservoir to move to the ampulla and meet the oocyte. There are several models to explain sperm release. One paradigm is that there is a controlled release of stored sperm near ovulation in response to a signal, perhaps from the ovulated oocyte or the released follicular fluid. There is an alternative hypothesis that a subset of sperm are released more stochastically at all times so that there is always a small number of sperm in the ampulla prepared to fertilize an oocyte. But even in this paradigm, it seems likely that there is some control over release of the heterogeneous population of sperm. Sperm release may be due to a change in the oviduct epithelial cells, in the sperm or in the fluid surrounding the cells.
The observation that capacitated sperm have reduced ability to bind oviduct glycans ( Kadirvel et al. , 2012 , Machado et al. , 2014 ) supports a model in which capacitation, the programmed maturation that sperm undergo, causes a change in oviduct glycan receptors so that sperm are released from the oviduct epithelium. Because capacitation does not occur synchronously, in this model sperm release would be expected to be stochastic. The mechanism behind this reduced binding during capacitation is not clear but it may be related to modification in the function of oviduct glycan receptors on sperm, perhaps by proteolysis. There is some preliminary evidence for proteolysis because one candidate glycan receptor, MFG-E8, co-precipitates in sperm lysates with a proteasomal subunit ( Miles et al. , 2013 ).
Another alternative is that the development of hyperactivated motility may be sufficient to detach a sperm from the oviduct epithelium ( Curtis et al. , 2012 ). In support of this, mouse sperm deficient in CatSper calcium channels that cannot hyperactivate do not detach from the oviduct ( Ho et al. , 2009 ).
There is evidence that the cumulus cells of the ovulated cumulus-oocyte complex can release chemical signals, such as progesterone ( Schoenfelder et al. , 2003 , Tosca et al. , 2007 ), which might activate localized sperm release by promoting Ca 2+ influx through CatSper channels ( Lishko et al. , 2012 ). Release may also be controlled by components from the oviduct itself, such as disulfide reducants ( Talevi et al. , 2007 , Brussow et al. , 2008 ), glycosidases that cleave oviduct glycans from the epithelium ( Carrasco et al. , 2008a , Carrasco et al. , 2008b ), and oviduct smooth muscle contractions ( Chang and Suarez, 2012 ). There is evidence that locally produced anandamide activates cannabinoid receptors and TRPV1 to induce a Ca 2+ influx and sperm release ( Gervasi et al. , 2016 ). Anandamide may also activate nitric oxide production by sperm to promote their release ( Osycka-Salut et al. , 2012 ). Finally, the production of unknown sulfated glyconjugates may release sperm by competing for binding sites on the oviduct epithelium ( Talevi and Gualtieri, 2010 ). The dynamic nature of sperm interaction with the oviduct suggests that a variety of factors may regulate sperm release that may aid in providing a constant supply of competent fertilizing sperm.
Immunological Tolerance of Sperm in the Oviduct
The oviduct lumen must maintain an aseptic state for successful fertilization and early embryonic development while regulating maternal responses to allogenic sperm and semi-allogenic embryos ( Marey et al. , 2016 ). Under pathologic conditions, the mucosal immune system produces a proinflammatory response. But sperm binding to oviduct epithelial cells induces an upregulation of IL-10 , TGFβ and increased production of prostaglandin E 2 , inducing an anti-inflammatory response ( Marey et al. , 2016 , Yousef et al. , 2016 ). This produces an environment that suppresses sperm phagocytosis by PMNs and allows sperm greater opportunity to survive in the oviduct and fertilize oocytes. In essence, sperm induce their own protection from an immune response in the oviduct.
Practical Applications of Unraveling the Complexities of Sperm-Female Tract Interaction
Understanding how the oviduct stores sperm should provide insight into how we could improve semen diluents to store sperm for longer periods of time without cryopreservation ( McGetrick et al. , 2014 ). This would be of great benefit to species whose sperm are stored as liquid semen for a few days because they do not survive cryopreservation. It would also benefit regions of the world that have poor infrastructure for storing cryopreserved semen (i.e. irregular delivery of liquid nitrogen) or use fresh semen routinely due to easy transportation and rapid use of the semen ( Vishwanath and Shannon, 2000 ). As proof of principle, addition of a specific soluble heat shock protein (HSPA8) to bull, boar and ram sperm can improve viability after a 24–48 hr incubation ( Elliott et al. , 2009 , Lloyd et al. , 2009 , Lloyd et al. , 2012 , Holt et al. , 2015 ).
A second application of this line of research is that it may lead to methods to lengthen sperm lifespan in the oviduct. This ability would improve fertility of females if semen deposition was not properly timed with,ovulation, a significant problem with AI in cattle and many other species. It may be possible to reduce the need for estrus detection in females because an accurate estimate of ovulation time might be less critical. Fertility despite the uncoupling of mating with ovulation has been accomplished by some mammals, notably some species of bats that store sperm for months, as well as snakes, reptiles and insects ( Holt and Fazeli, 2016 ). Although the opportunity to reduce estrus detection by lengthening sperm lifespan may be overly optimistic, the examples in nature of species that store sperm for a long duration suggest that it may be possible.

Implications
Sperm interaction with the cow reproductive tract after semen deposition has a profound influence on pregnancy rates and provides perplexing fundamental questions that are unresolved despite considerable study. The fertilizing sperm are selected by the tract from the millions or billions of sperm deposited at mating or artificial insemination. Successful sperm interact with luminal fluid and epithelia, while evading destruction by the immune system. They respond to rheotactic, chemical and adhesive stimuli to undergo functional changes and arrive at the site of fertilization. An understanding of how these processes are coordinated can improve in vitro fertilization success, contraception effectiveness, sperm lifespan in the oviduct, improved semen storage, and fertility.
Acknowledgements
Work in the author’s laboratory was supported by Agriculture and Food Research Initiative Competitive Grant no. 2011–67015–20099 and 2015–67015–23228 from the USDA National Institute of Food and Agriculture and the National Science Foundation. The author acknowledges Rebecca Winters and Lantana Grub for comments that improved the manuscript and for being unable to discuss other important work due to length restrictions.
Conflict of Interest
There are no conflicts of interest.
List of References
- Abe H, Sendai Y, Satoh T and Hoshi H 1995. Bovine oviduct-specific glycoprotein: A potent factor for maintenance of viability and motility of bovine spermatozoa in vitro . Molecular Reproduction and Development 42 , 226–232. [ PubMed ] [ Google Scholar ]
- Al-Dossary AA, Bathala P, Caplan JL and Martin-DeLeon PA 2015. Oviductosome-sperm membrane interaction in cargo delivery: Detection of fusion and underlying molecular players using three-dimensional super-resolution structured illumination microscopy (sr-sim) . Journal of Biological Chemistry 290 , 17710–17723. [ PMC free article ] [ PubMed ] [ Google Scholar ]
- Alasmari W, Costello S, Correia J, Oxenham SK, Morris J, Fernandes L, Ramalho-Santos J, Kirkman-Brown J, Michelangeli F, Publicover S and Barratt CL 2013. Ca2+ signals generated by catsper and ca2+ stores regulate different behaviors in human sperm . Journal of Biological Chemistry 288 , 6248–6258. [ PMC free article ] [ PubMed ] [ Google Scholar ]
- Algarra B, Han L, Soriano-Ubeda C, Aviles M, Coy P, Jovine L and Jimenez-Movilla M 2016. The c-terminal region of ovgp1 remodels the zona pellucida and modifies fertility parameters . Scientific Reports 6 , 32556. [ PMC free article ] [ PubMed ] [ Google Scholar ]
- Alminana C, Caballero I, Heath PR, Maleki-Dizaji S, Parrilla I, Cuello C, Gil MA, Vazquez JL, Vazquez JM, Roca J, Martinez EA, Holt WV and Fazeli A 2014. The battle of the sexes starts in the oviduct: Modulation of oviductal transcriptome by x and y-bearing spermatozoa . BMC Genomics 15 , 293. [ PMC free article ] [ PubMed ] [ Google Scholar ]
- Amann RP and Griel LC Jr. 1974. Fertility of bovine spermatozoa from rete testis, cauda epididymidis, and ejaculated semen . Journal of Dairy Science 57 , 212–219. [ PubMed ] [ Google Scholar ]
- Araki Y, Nohara M, Yoshida-Komiya H, Kuramochi T, Ito M, Hoshi H, Shinkai Y and Sendai Y 2003. Effect of a null mutation of the oviduct-specific glycoprotein gene on mouse fertilization . Biochemical Journal 374 , 551–557. [ PMC free article ] [ PubMed ] [ Google Scholar ]
- Atikuzzaman M, Alvarez-Rodriguez M, Vicente-Carrillo A, Johnsson M, Wright D and Rodriguez-Martinez H 2017. Conserved gene expression in sperm reservoirs between birds and mammals in response to mating . BMC Genomics 18 , 98. [ PMC free article ] [ PubMed ] [ Google Scholar ]
- Bergqvist AS, Yokoo M, Bage R, Sato E and Rodriguez-Martinez H 2005a. Detection of the hyaluronan receptor cd44 in the bovine oviductal epithelium . Journal of Reproduction and Development 51 , 445–453. [ PubMed ] [ Google Scholar ]
- Bergqvist AS, Ballester J, Johannisson A, Lundeheim N and Rodriguez-Martinez H 2007. Heparin and dermatan sulphate induced capacitation of frozen-thawed bull spermatozoa measured by merocyanine-540 . Zygote 15 , 225–232. [ PubMed ] [ Google Scholar ]
- Bergqvist AS, Yokoo M, Heldin P, Frendin J, Sato E and Rodriguez-Martinez H 2005b. Hyaluronan and its binding proteins in the epithelium and intraluminal fluid of the bovine oviduct . Zygote 13 , 207–218. [ PubMed ] [ Google Scholar ]
- Bergqvist AS, Ballester J, Johannisson A, Hernandez M, Lundeheim N and Rodriguez-Martinez H 2006. In vitro capacitation of bull spermatozoa by oviductal fluid and its components . Zygote 14 , 259–273. [ PubMed ] [ Google Scholar ]
- Boilard M, Bailey J, Collin S, Dufour M and Sirard MA 2002. Effect of bovine oviduct epithelial cell apical plasma membranes on sperm function assessed by a novel flow cytometric approach . Biology of Reproduction 67 , 1125–1132. [ PubMed ] [ Google Scholar ]
- Boilard M, Reyes-Moreno C, Lachance C, Massicotte L, Bailey JL, Sirard MA and Leclerc P 2004. Localization of the chaperone proteins grp78 and hsp60 on the luminal surface of bovine oviduct epithelial cells and their association with spermatozoa . Biology of Reproduction 71 , 1879–1889. [ PubMed ] [ Google Scholar ]
- Bromfield JJ 2014. Seminal fluid and reproduction: Much more than previously thought . Journal of Assist Reproduction and Genetics 31 , 627–636. [ PMC free article ] [ PubMed ] [ Google Scholar ]
- Bromfield JJ, Schjenken JE, Chin PY, Care AS, Jasper MJ and Robertson SA 2014. Maternal tract factors contribute to paternal seminal fluid impact on metabolic phenotype in offspring . Proceedings of the National Academy of Sciences USA 111 , 2200–2205. [ PMC free article ] [ PubMed ] [ Google Scholar ]
- Brussow KP, Ratky J and Rodriguez-Martinez H 2008. Fertilization and early embryonic development in the porcine fallopian tube . Reproduction in Domestic Animals 43 Suppl 2 , 245–251. [ PubMed ] [ Google Scholar ]
- Caballero JN, Gervasi MG, Veiga MF, Dalvit GC, Perez-Martinez S, Cetica PD and Vazquez-Levin MH 2014. Epithelial cadherin is present in bovine oviduct epithelial cells and gametes, and is involved in fertilization-related events . Theriogenology 81 , 1189–1206. [ PubMed ] [ Google Scholar ]
- Carrasco LC, Coy P, Aviles M, Gadea J and Romar R 2008a. Glycosidase determination in bovine oviducal fluid at the follicular and luteal phases of the oestrous cycle . Reproduction Fertility and Development 20 , 808–817. [ PubMed ] [ Google Scholar ]
- Carrasco LC, Romar R, Aviles M, Gadea J and Coy P 2008b. Determination of glycosidase activity in porcine oviductal fluid at the different phases of the estrous cycle . Reproduction 136 , 833–842. [ PubMed ] [ Google Scholar ]
- Chang H and Suarez SS 2012. Unexpected flagellar movement patterns and epithelial binding behavior of mouse sperm in the oviduct . Biology of Reproduction 86 , 140 , 1–8. [ PMC free article ] [ PubMed ] [ Google Scholar ]
- Christoffersen M and Troedsson M 2017. Inflammation and fertility in the mare . Reproduction in Domestic Animals 52 Suppl 3 , 14–20. [ PubMed ] [ Google Scholar ]
- Cortes PP, Orihuela PA, Zuniga LM, Velasquez LA and Croxatto HB 2004. Sperm binding to oviductal epithelial cells in the rat: Role of sialic acid residues on the epithelial surface and sialic acid-binding sites on the sperm surface . Biology of Reproduction 71 , 1262–1269. [ PubMed ] [ Google Scholar ]
- Coy P, Lloyd R, Romar R, Satake N, Matas C, Gadea J and Holt WV 2010. Effects of porcine pre-ovulatory oviductal fluid on boar sperm function . Theriogenology 74 , 632–642. [ PubMed ] [ Google Scholar ]
- Coy P, Canovas S, Mondejar I, Saavedra MD, Romar R, Grullon L, Matas C and Aviles M 2008. Oviduct-specific glycoprotein and heparin modulate sperm-zona pellucida interaction during fertilization and contribute to the control of polyspermy . Proceedings of the National Academy of Sciences USA 105 , 15809–15814. [ PMC free article ] [ PubMed ] [ Google Scholar ]
- Curtis MP, Kirkman-Brown JC, Connolly TJ and Gaffney EA 2012. Modelling a tethered mammalian sperm cell undergoing hyperactivation . J Theor Biol 309C , 1–10. [ PubMed ] [ Google Scholar ]
- DeJarnette JM, Nebel RL and Marshall CE 2009. Evaluating the success of sex-sorted semen in us dairy herds from on farm records . Theriogenology 71 , 49–58. [ PubMed ] [ Google Scholar ]
- Demott RP and Suarez SS 1992. Hyperactivated sperm progress in the mouse oviduct . Biology of Reproduction 46 , 779–785. [ PubMed ] [ Google Scholar ]
- Denissenko P, Kantsler V, Smith DJ and Kirkman-Brown J 2012. Human spermatozoa migration in microchannels reveals boundary-following navigation . Proceedings of the National Academy of Sciences USA 109 , 8007–8010. [ PMC free article ] [ PubMed ] [ Google Scholar ]
- Dobrinski I, Ignotz GG, Thomas PG and Ball BA 1996. Role of carbohydrates in the attachment of equine spermatozoa to uterine tubal (oviductal) epithelial cells in vitro . American Journal of Veterinary Research 57 , 1635–1639. [ PubMed ] [ Google Scholar ]
- Dobrinski I, Smith TT, Suarez SS and Ball BA 1997. Membrane contact with oviductal epithelium modulates the intracellular calcium concentration of equine spermatozoa in vitro . Biology of Reproduction 56 , 861–869. [ PubMed ] [ Google Scholar ]
- Ehrenwald E, Foote RH and Parks JE 1990. Bovine oviductal fluid components and their potential role in sperm cholesterol efflux . Molecular Reproduction and Development 25 , 195–204. [ PubMed ] [ Google Scholar ]
- Ekhlasi-Hundrieser M, Gohr K, Wagner A, Tsolova M, Petrunkina A and Topfer-Petersen E 2005. Spermadhesin aqn1 is a candidate receptor molecule involved in the formation of the oviductal sperm reservoir in the pig . Biology of Reproduction 73 , 536–545. [ PubMed ] [ Google Scholar ]
- El-Sherry TM, Elsayed M, Abdelhafez HK and Abdelgawad M 2014. Characterization of rheotaxis of bull sperm using microfluidics . Integrative Biology (Cambridge) 6 , 1111–1121. [ PubMed ] [ Google Scholar ]
- Elliott RM, Lloyd RE, Fazeli A, Sostaric E, Georgiou AS, Satake N, Watson PF and Holt WV 2009. Effects of hspa8, an evolutionarily conserved oviductal protein, on boar and bull spermatozoa . Reproduction 137 , 191–203. [ PubMed ] [ Google Scholar ]
- Erikson DW, Way AL, Chapman DA and Killian GJ 2007. Detection of osteopontin on holstein bull spermatozoa, in cauda epididymal fluid and testis homogenates, and its potential role in bovine fertilization . Reproduction 133 , 909–917. [ PubMed ] [ Google Scholar ]
- Evans RW and Setchell BP 1978. Association of exogenous phospholipids with spermatozoa . Journal of Reproduction and Fertility 53 , 357–362. [ PubMed ] [ Google Scholar ]
- Fazeli A, Affara NA, Hubank M and Holt WV 2004. Sperm-induced modification of the oviductal gene expression profile after natural insemination in mice . Biology of Reproduction 71 , 60–65. [ PubMed ] [ Google Scholar ]
- Fazeli A, Elliott RM, Duncan AE, Moore A, Watson PF and Holt WV 2003. In vitro maintenance of boar sperm viability by a soluble fraction obtained from oviductal apical plasma membrane preparations . Reproduction 125 , 509–517. [ PubMed ] [ Google Scholar ]
- Georgiou AS, Sostaric E, Wong CH, Snijders AP, Wright PC, Moore HD and Fazeli A 2005. Gametes alter the oviductal secretory proteome . Molecular and Cellular Proteomics 4 , 1785–1796. [ PubMed ] [ Google Scholar ]
- Georgiou AS, Snijders AP, Sostaric E, Aflatoonian R, Vazquez JL, Vazquez JM, Roca J, Martinez EA, Wright PC and Fazeli A 2007. Modulation of the oviductal environment by gametes . Journal of Proteome Research 6 , 4656–4666. [ PubMed ] [ Google Scholar ]
- Gervasi MG, Osycka-Salut C, Sanchez T, Alonso CA, Llados C, Castellano L, Franchi AM, Villalon M and Perez-Martinez S 2016. Sperm release from the oviductal epithelium depends on ca(2+) influx upon activation of cb1 and trpv1 by anandamide . Journal of Cellular Biochemistry 117 , 320–333. [ PubMed ] [ Google Scholar ]
- Goncalves RF, Chapman DA, Bertolla RP, Eder I and Killian GJ 2008. Pre-treatment of cattle semen or oocytes with purified milk osteopontin affects in vitro fertilization and embryo development . Animal Reproduction Science 108 , 375–383. [ PubMed ] [ Google Scholar ]
- Gonzalez-Abreu D, Garcia-Martinez S, Fernandez-Espin V, Romar R and Gadea J 2017. Incubation of boar spermatozoa in viscous media by addition of methylcellulose improves sperm quality and penetration rates during in vitro fertilization . Theriogenology 92 , 14–23. [ PubMed ] [ Google Scholar ]
- Green CE, Bredl J, Holt WV, Watson PF and Fazeli A 2001. Carbohydrate mediation of boar sperm binding to oviductal epithelial cells in vitro . Reproduction 122 , 305–315. [ PubMed ] [ Google Scholar ]
- Hansen PJ 2011. The immunology of early pregnancy in farm animals . Reproduction in Domestic Animals 46 Suppl 3 , 18–30. [ PubMed ] [ Google Scholar ]
- Hawk HW 1987. Transport and fate of spermatozoa after insemination of cattle . Journal of Dairy Science 70 , 1487–1503. [ PubMed ] [ Google Scholar ]
- Ho K, Wolff CA and Suarez SS 2009. Catsper-null mutant spermatozoa are unable to ascend beyond the oviductal reservoir . Reproduction Fertility and Development 21 , 345–350. [ PubMed ] [ Google Scholar ]
- Holt WV and Fazeli A 2016. Sperm storage in the female reproductive tract . Annual Reviews in Animal Biosciences 4 , 291–310. [ PubMed ] [ Google Scholar ]
- Holt WV, Del Valle I and Fazeli A 2015. Heat shock protein a8 stabilizes the bull sperm plasma membrane during cryopreservation: Effects of breed, protein concentration, and mode of use . Theriogenology 84 , 693–701. [ PubMed ] [ Google Scholar ]
- Hung PH and Suarez SS 2010. Regulation of sperm storage and movement in the ruminant oviduct . Society of Reproduction and Fertility Suppl 67 , 257–266. [ PubMed ] [ Google Scholar ]
- Hunter RH 1995. Significance of the epithelial crypts at the bovine utero-tubal junction in the pre-ovulatory phase of sperm regulation . Acta Veterinaria Scandinavica 36 , 413–421. [ PMC free article ] [ PubMed ] [ Google Scholar ]
- Hunter RH 2002. Vital aspects of fallopian tube physiology in pigs . Reproduction in Domestic Animals 37 , 186–190. [ PubMed ] [ Google Scholar ]
- Hunter RH and Leglise PC 1971a. Tubal surgery in the rabbit: Fertilization and polyspermy after resection of the isthmus . American Journal of Anatomy 132 , 45–52. [ PubMed ] [ Google Scholar ]
- Hunter RH and Leglise PC 1971b. Polyspermic fertilization following tubal surgery in pigs, with particular reference to the role of the isthmus . Journal of Reproduction and Fertility 24 , 233–246. [ PubMed ] [ Google Scholar ]
- Hunter RH, Nichol R and Crabtree SM 1980. Transport of spermatozoa in the ewe: Timing of the establishment of a functional population in the oviduct . Reproduction Nutrition and Development 20 , 1869–1875. [ PubMed ] [ Google Scholar ]
- Hyakutake T, Suzuki H and Yamamoto S 2015. Effect of non-newtonian fluid properties on bovine sperm motility . Journal of Biomechanics 48 , 2941–2947. [ PubMed ] [ Google Scholar ]
- Ignotz GG, Cho MY and Suarez SS 2007. Annexins are candidate oviductal receptors for bovine sperm surface proteins and thus may serve to hold bovine sperm in the oviductal reservoir . Biology of Reproduction 77 , 906–913. [ PubMed ] [ Google Scholar ]
- Ishikawa Y, Usui T, Yamashita M, Kanemori Y and Baba T 2016. Surfing and swimming of ejaculated sperm in the mouse oviduct . Biology of Reproduction 94 , 89. [ PubMed ] [ Google Scholar ]
- Jaffe RC, Arias EB, O’Day-Bowman MB, Donnelly KM, Mavrogianis PA and Verhage HG 1996. Regional distribution and hormonal control of estrogen-dependent oviduct-specific glycoprotein messenger ribonucleic acid in the baboon (papio anubis) . Biology of Reproduction 55 , 421–426. [ PubMed ] [ Google Scholar ]
- Jansen RP 1978. Fallopian tube isthmic mucus and ovum transport . Science 201 , 349–351. [ PubMed ] [ Google Scholar ]
- Jin M, Fujiwara E, Kakiuchi Y, Okabe M, Satouh Y, Baba SA, Chiba K and Hirohashi N 2011. Most fertilizing mouse spermatozoa begin their acrosome reaction before contact with the zona pellucida during in vitro fertilization . Proceedings of the National Academy of Sciences USA 108 , 4892–4896. [ PMC free article ] [ PubMed ] [ Google Scholar ]
- Kadirvel G, Machado SA, Korneli C, Collins E, Miller P, Bess KN, Aoki K, Tiemeyer M, Bovin N and Miller DJ 2012. Porcine sperm bind to specific 6-sialylated biantennary glycans to form the oviduct reservoir . Biology of Reproduction 87 , 147. [ PMC free article ] [ PubMed ] [ Google Scholar ]
- Katila T 2012. Post-mating inflammatory responses of the uterus . Reproduction in Domestic Animals 47 Suppl 5 , 31–41. [ PubMed ] [ Google Scholar ]
- Katz DF, Slade DA and Nakajima ST 1997. Analysis of pre-ovulatory changes in cervical mucus hydration and sperm penetrability . Advances in Contraception 13 , 143–151. [ PubMed ] [ Google Scholar ]
- Kawano N, Araki N, Yoshida K, Hibino T, Ohnami N, Makino M, Kanai S, Hasuwa H, Yoshida M, Miyado K and Umezawa A 2014. Seminal vesicle protein svs2 is required for sperm survival in the uterus . Proceedings of the National Academy of Sciences USA 111 , 4145–4150. [ PMC free article ] [ PubMed ] [ Google Scholar ]
- Kervancioglu ME, Saridogan E, Aitken RJ and Djahanbakhch O 2000. Importance of sperm-to-epithelial cell contact for the capacitation of human spermatozoa in fallopian tube epithelial cell cocultures . Fertility and Sterility 74 , 780–784. [ PubMed ] [ Google Scholar ]
- Killian G 2011. Physiology and endocrinology symposium: Evidence that oviduct secretions influence sperm function: A retrospective view for livestock . Journal of Animal Science 89 , 1315–1322. [ PubMed ] [ Google Scholar ]
- Killian GJ, Chapman DA, Kavanaugh JF, Deaver DR and Wiggin HB 1989. Changes in phospholipids, cholesterol and protein content of oviduct fluid of cows during the oestrous cycle . Journal of Reproduction and Fertility 86 , 419–426. [ PubMed ] [ Google Scholar ]
- Kirkman-Brown JC and Smith DJ 2011. Sperm motility: Is viscosity fundamental to progress? Molecular Human Reproduction 17 , 539–544. [ PubMed ] [ Google Scholar ]
- Kunz G, Beil D, Deininger H, Wildt L and Leyendecker G 1996. The dynamics of rapid sperm transport through the female genital tract: Evidence from vaginal sonography of uterine peristalsis and hysterosalpingoscintigraphy . Human Reproduction 11 , 627–632. [ PubMed ] [ Google Scholar ]
- Kutteh WH, Prince SJ, Hammond KR, Kutteh CC and Mestecky J 1996. Variations in immunoglobulins and iga subclasses of human uterine cervical secretions around the time of ovulation . Clinical and Experimental Immunology 104 , 538–542. [ PMC free article ] [ PubMed ] [ Google Scholar ]
- La Spina FA, Puga Molina LC, Romarowski A, Vitale AM, Falzone TL, Krapf D, Hirohashi N and Buffone MG 2016. Mouse sperm begin to undergo acrosomal exocytosis in the upper isthmus of the oviduct . Developmental Biology 411 , 172–182. [ PMC free article ] [ PubMed ] [ Google Scholar ]
- Lamy J, Labas V, Harichaux G, Tsikis G, Mermillod P and Saint-Dizier M 2016. Regulation of the bovine oviductal fluid proteome . Reproduction 152 , 629–644. [ PubMed ] [ Google Scholar ]
- Lapointe J and Bilodeau JF 2003. Antioxidant defenses are modulated in the cow oviduct during the estrous cycle . Biology of Reproduction 68 , 1157–1164. [ PubMed ] [ Google Scholar ]
- Lefebvre R, Lo MC and Suarez SS 1997. Bovine sperm binding to oviductal epithelium involves fucose recognition . Biology of Reproduction 56 , 1198–1204. [ PubMed ] [ Google Scholar ]
- Lefebvre R, Chenoweth PJ, Drost M, LeClear CT, MacCubbin M, Dutton JT and Suarez SS 1995. Characterization of the oviductal sperm reservoir in cattle . Biology of Reproduction 53 , 1066–1074. [ PubMed ] [ Google Scholar ]
- Lishko PV, Kirichok Y, Ren D, Navarro B, Chung JJ and Clapham DE 2012. The control of male fertility by spermatozoan ion channels . Annual Reviews in Physiology 74 , 453–475. [ PMC free article ] [ PubMed ] [ Google Scholar ]
- Lloyd RE, Fazeli A, Watson PF and Holt WV 2012. The oviducal protein, heat-shock 70-kda protein 8, improves the long-term survival of ram spermatozoa during storage at 17 degrees c in a commercial extender . Reproduction Fertility and Development 24 , 543–549. [ PubMed ] [ Google Scholar ]
- Lloyd RE, Elliott RM, Fazeli A, Watson PF and Holt WV 2009. Effects of oviductal proteins, including heat shock 70 kda protein 8, on survival of ram spermatozoa over 48 h in vitro . Reproduction Fertility and Development 21 , 408–418. [ PubMed ] [ Google Scholar ]
- Lopez-Ubeda R, Munoz M, Vieira L, Hunter RH, Coy P and Canovas S 2016. The oviductal transcriptome is influenced by a local ovarian effect in the sow . Journal of Ovarian Research 9 , 44. [ PMC free article ] [ PubMed ] [ Google Scholar ]
- Lopez-Ubeda R, Garcia-Vazquez FA, Romar R, Gadea J, Munoz M, Hunter RH and Coy P 2015. Oviductal transcriptome is modified after insemination during spontaneous ovulation in the sow . PLoS ONE 10 , e0130128. [ PMC free article ] [ PubMed ] [ Google Scholar ]
- Lovell JW and Getty R 1968. Fate of semen in the uterus of the sow: Histologic study of endometrium during the 27 hours after natural service . American Journal of Veterinary Research 29 , 609–625. [ PubMed ] [ Google Scholar ]
- Lyng R and Shur BD 2009. Mouse oviduct-specific glycoprotein is an egg-associated zp3-independent sperm-adhesion ligand . Journal of Cell Science 122 , 3894–3906. [ PMC free article ] [ PubMed ] [ Google Scholar ]
- Machado SA, Kadirvel G, Daigneault BW, Korneli C, Miller P, Bovin N and Miller DJ 2014. Lewisx-containing glycans on the porcine oviductal epithelium contribute to formation of the sperm reservoir . Biology of Reproduction 91 , 140. [ PubMed ] [ Google Scholar ]
- Marey MA, Yousef MS, Kowsar R, Hambruch N, Shimizu T, Pfarrer C and Miyamoto A 2016. Local immune system in oviduct physiology and pathophysiology: Attack or tolerance? Domest Animal Endocrinology 56 Suppl , S204–211. [ PubMed ] [ Google Scholar ]
- Mathur S, Rosenlund C, Carlton M, Caldwell J, Barber M, Rust PF and Williamson HO 1988. Studies on sperm survival and motility in the presence of cytotoxic sperm antibodies . American Journal of Reproductive Immunology and Microbiology 17 , 41–47. [ PubMed ] [ Google Scholar ]
- McGetrick JA, Reid CJ and Carrington SD 2014. Improving bovine semen diluents: Insights from the male and female reproductive tracts, and the potential relevance of cervical mucins . Animal 8 Suppl 1 , 173–184. [ PubMed ] [ Google Scholar ]
- Miki K and Clapham DE 2013. Rheotaxis guides mammalian sperm . Current Biology 23 , 443–452. [ PMC free article ] [ PubMed ] [ Google Scholar ]
- Miles EL, O’Gorman C, Zhao J, Samuel M, Walters E, Yi YJ, Sutovsky M, Prather RS, Wells KD and Sutovsky P 2013. Transgenic pig carrying green fluorescent proteasomes . Proceedings of the National Academy of Sciences USA 110 , 6334–6339. [ PMC free article ] [ PubMed ] [ Google Scholar ]
- Moore SG and Hasler JF 2017. A 100-year review: Reproductive technologies in dairy science . Journal of Dairy Science 100 , 10314–10331. [ PubMed ] [ Google Scholar ]
- Mullins KJ and Saacke RG 1989. Study of the functional anatomy of bovine cervical mucosa with special reference to mucus secretion and sperm transport . Anatomical Record 225 , 106–117. [ PubMed ] [ Google Scholar ]
- Muro Y, Hasuwa H, Isotani A, Miyata H, Yamagata K, Ikawa M, Yanagimachi R and Okabe M 2016. Behavior of mouse spermatozoa in the female reproductive tract from soon after mating to the beginning of fertilization . Biology of Reproduction 94 , 80. [ PubMed ] [ Google Scholar ]
- Murray SC and Smith TT 1997. Sperm interaction with fallopian tube apical membrane enhances sperm motility and delays capacitation . Fertility and Sterility 68 , 351–357. [ PubMed ] [ Google Scholar ]
- Naito Y, Takematsu H, Koyama S, Miyake S, Yamamoto H, Fujinawa R, Sugai M, Okuno Y, Tsujimoto G, Yamaji T, Hashimoto Y, Itohara S, Kawasaki T, Suzuki A and Kozutsumi Y 2007. Germinal center marker gl7 probes activation-dependent repression of n-glycolylneuraminic acid, a sialic acid species involved in the negative modulation of b-cell activation . Molecular and Cellular Biology 27 , 3008–3022. [ PMC free article ] [ PubMed ] [ Google Scholar ]
- Nakanishi T, Isotani A, Yamaguchi R, Ikawa M, Baba T, Suarez SS and Okabe M 2004. Selective passage through the uterotubal junction of sperm from a mixed population produced by chimeras of calmegin-knockout and wild-type male mice . Biology of Reproduction 71 , 959–965. [ PubMed ] [ Google Scholar ]
- Okabe M 2013. The cell biology of mammalian fertilization . Development 140 , 4471–4479. [ PubMed ] [ Google Scholar ]
- Orr TJ and Zuk M 2014. Reproductive delays in mammals: An unexplored avenue for post-copulatory sexual selection . Biological Reviews of the Cambridge Philosophical Society 89 , 889–912. [ PubMed ] [ Google Scholar ]
- Osycka-Salut C, Gervasi MG, Pereyra E, Cella M, Ribeiro ML, Franchi AM and Perez-Martinez S 2012. Anandamide induces sperm release from oviductal epithelia through nitric oxide pathway in bovines . PLoS ONE 7 , e30671. [ PMC free article ] [ PubMed ] [ Google Scholar ]
- Osycka-Salut CE, Castellano L, Fornes D, Beltrame JS, Alonso CAI, Jawerbaum A, Franchi A, Diaz ES and Perez Martinez S 2017. Fibronectin from oviductal cells fluctuates during the estrous cycle and contributes to sperm-oviduct interaction in cattle . Journal of Cellular Biochemistry 118 , 4095–4108. [ PubMed ] [ Google Scholar ]
- Pacey AA, Hill CJ, Scudamore IW, Warren MA, Barratt CL and Cooke ID 1995. The interaction in vitro of human spermatozoa with epithelial cells from the human uterine (fallopian) tube . Human Reproduction 10 , 360–366. [ PubMed ] [ Google Scholar ]
- Parrish JJ, Susko-Parrish JL, Handrow RR, Sims MM and First NL 1989. Capacitation of bovine spermatozoa by oviduct fluid . Biology of Reproduction 40 , 1020–1025. [ PubMed ] [ Google Scholar ]
- Petrunkina AM, Gehlhaar R, Drommer W, Waberski D and Topfer-Petersen E 2001. Selective sperm binding to pig oviductal epithelium in vitro . Reproduction 121 , 889–896. [ PubMed ] [ Google Scholar ]
- Pillai VV, Weber DM, Phinney BS and Selvaraj V 2017. Profiling of proteins secreted in the bovine oviduct reveals diverse functions of this luminal microenvironment . PLoS ONE 12 , e0188105. [ PMC free article ] [ PubMed ] [ Google Scholar ]
- Pollard JW, Plante C, King WA, Hansen PJ, Betteridge KJ and Suarez SS 1991. Fertilizing capacity of bovine sperm may be maintained by binding of oviductal epithelial cells . Biology of Reproduction 44 , 102–107. [ PubMed ] [ Google Scholar ]
- Rath D, Knorr C and Taylor U 2016. Communication requested: Boar semen transport through the uterus and possible consequences for insemination . Theriogenology 85 , 94–104. [ PubMed ] [ Google Scholar ]
- Rijsselaere T, Van Soom A, Van Cruchten S, Coryn M, Gortz K, Maes D and de Kruif A 2004. Sperm distribution in the genital tract of the bitch following artificial insemination in relation to the time of ovulation . Reproduction 128 , 801–811. [ PubMed ] [ Google Scholar ]
- Rittling SR, Matsumoto HN, McKee MD, Nanci A, An XR, Novick KE, Kowalski AJ, Noda M and Denhardt DT 1998. Mice lacking osteopontin show normal development and bone structure but display altered osteoclast formation in vitro . Journal of Bone and Mineral Research 13 , 1101–1111. [ PubMed ] [ Google Scholar ]
- Robertson SA 2007. Seminal fluid signaling in the female reproductive tract: Lessons from rodents and pigs . Journal of Animal Science 85 , E36–44. [ PubMed ] [ Google Scholar ]
- Rodriguez-Martinez H 2007. Role of the oviduct in sperm capacitation . Theriogenology 68 Suppl 1 , S138–146. [ PubMed ] [ Google Scholar ]
- Rodriguez-Martinez H, Saravia F, Wallgren M, Tienthai P, Johannisson A, Vazquez JM, Martinez E, Roca J, Sanz L and Calvete JJ 2005. Boar spermatozoa in the oviduct . Theriogenology 63 , 514–535. [ PubMed ] [ Google Scholar ]
- Rutllant J, Lopez-Bejar M and Lopez-Gatius F 2005. Ultrastructural and rheological properties of bovine vaginal fluid and its relation to sperm motility and fertilization: A review . Reproduction in Domestic Animals 40 , 79–86. [ PubMed ] [ Google Scholar ]
- Sanz L, Calvete JJ, Mann K, Gabius HJ and Topfer-Petersen E 1993. Isolation and biochemical characterization of heparin-binding proteins from boar seminal plasma: A dual role for spermadhesins in fertilization . Molecular Reproduction and Development 35 , 37–43. [ PubMed ] [ Google Scholar ]
- Schjenken JE and Robertson SA 2014. Seminal fluid and immune adaptation for pregnancy--comparative biology in mammalian species . Reproduction in Domestic Animals 49 Suppl 3 , 27–36. [ PubMed ] [ Google Scholar ]
- Schjenken JE and Robertson SA 2015. Seminal fluid signalling in the female reproductive tract: Implications for reproductive success and offspring health . Advances in Experimental Medicine and Biology 868 , 127–158. [ PubMed ] [ Google Scholar ]
- Schoenfelder M, Schams D and Einspanier R 2003. Steroidogenesis during in vitro maturation of bovine cumulus oocyte complexes and possible effects of tri-butyltin on granulosa cells . Journal of Steroid Biochemistry and Molecular Biology 84 , 291–300. [ PubMed ] [ Google Scholar ]
- Silva E, Kadirvel G, Jiang R, Bovin N and Miller D 2014. Multiple proteins from ejaculated and epididymal porcine spermatozoa bind glycan motifs found in the oviduct . Andrology 2 , 763–771. [ PubMed ] [ Google Scholar ]
- Silva E, Frost D, Li L, Bovin N and Miller DJ 2017. Lactadherin is a candidate oviduct lewis x trisaccharide receptor on porcine spermatozoa . Andrology 5 , 589–597. [ PMC free article ] [ PubMed ] [ Google Scholar ]
- Smith TT and Nothnick WB 1997. Role of direct contact between spermatozoa and oviductal epithelial cells in maintaining rabbit sperm viability . Biology of Reproduction 56 , 83–89. [ PubMed ] [ Google Scholar ]
- Song X, Yu H, Chen X, Lasanajak Y, Tappert MM, Air GM, Tiwari VK, Cao H, Chokhawala HA, Zheng H, Cummings RD and Smith DF 2011. A sialylated glycan microarray reveals novel interactions of modified sialic acids with proteins and viruses . Journal of Biological Chemistry 286 , 31610–31622. [ PMC free article ] [ PubMed ] [ Google Scholar ]
- Suarez S, Redfern K, Raynor P, Martin F and Phillips DM 1991. Attachment of boar sperm to mucosal explants of oviduct in vitro: Possible role in formation of a sperm reservoir . Biology of Reproduction 44 , 998–1004. [ PubMed ] [ Google Scholar ]
- Suarez SS 2001. Carbohydrate-mediated formation of the oviductal sperm reservoir in mammals . Cells Tissues Organs 168 , 105–112. [ PubMed ] [ Google Scholar ]
- Suarez SS and Dai X 1992. Hyperactivation enhances mouse sperm capacity for penetrating viscoelastic media . Biology of Reproduction 46 , 686–691. [ PubMed ] [ Google Scholar ]
- Suarez SS and Pacey AA 2006. Sperm transport in the female reproductive tract . Human Reproduction Update 12 , 23–37. [ PubMed ] [ Google Scholar ]
- Suarez SS, Brockman K and Lefebvre R 1997. Distribution of mucus and sperm in bovine oviducts after artificial insemination: The physical environment of the oviductal sperm reservoir . Biology of Reproduction 56 , 447–453. [ PubMed ] [ Google Scholar ]
- Suarez SS, Revah I, Lo M and Kolle S 1998. Bull sperm binding to oviductal epithelium is mediated by a ca2+-dependent lectin on sperm that recognizes lewis-a trisaccharide . Biology of Reproduction 59 , 39–44. [ PubMed ] [ Google Scholar ]
- Talevi R and Gualtieri R 2010. Molecules involved in sperm-oviduct adhesion and release . Theriogenology 73 , 796–801. [ PubMed ] [ Google Scholar ]
- Talevi R, Zagami M, Castaldo M and Gualtieri R 2007. Redox regulation of sperm surface thiols modulates adhesion to the fallopian tube epithelium . Biology of Reproduction 76 , 728–735. [ PubMed ] [ Google Scholar ]
- Teijeiro JM and Marini PE 2012. Apical membranes prepared by peeling from whole porcine oviducts interact with homologous sperm . Cell and Tissue Research 348 , 213–223. [ PubMed ] [ Google Scholar ]
- Teijeiro JM, Dapino DG and Marini PE 2011. Porcine oviduct sperm binding glycoprotein and its deleterious effect on sperm: A mechanism for negative selection of sperm? Biological Research 44 , 329–337. [ PubMed ] [ Google Scholar ]
- Thomas PG, Ball BA and Brinsko SP 1994. Interaction of equine spermatozoa with oviduct epithelial cell explants is affected by estrous cycle and anatomic origin of explant . Biology of Reproduction 51 , 222–228. [ PubMed ] [ Google Scholar ]
- Tienthai P 2015. The porcine sperm reservoir in relation to the function of hyaluronan . Journal of Reproduction and Development 61 , 245–250. [ PMC free article ] [ PubMed ] [ Google Scholar ]
- Topfer-Petersen E, Ekhlasi-Hundrieser M and Tsolova M 2008. Glycobiology of fertilization in the pig . International Journal of Developmental Biology 52 , 717–736. [ PubMed ] [ Google Scholar ]
- Tosca L, Uzbekova S, Chabrolle C and Dupont J 2007. Possible role of 5’amp-activated protein kinase in the metformin-mediated arrest of bovine oocytes at the germinal vesicle stage during in vitro maturation . Biology of Reproduction 77 , 452–465. [ PubMed ] [ Google Scholar ]
- Tung CK, Ardon F, Roy A, Koch DL, Suarez SS and Wu M 2015a. Emergence of upstream swimming via a hydrodynamic transition . Physical Review Letters 114 , 108102. [ PMC free article ] [ PubMed ] [ Google Scholar ]
- Tung CK, Lin C, Harvey B, Fiore AG, Ardon F, Wu M and Suarez SS 2017. Fluid viscoelasticity promotes collective swimming of sperm . Scientific Reports 7 , 3152. [ PMC free article ] [ PubMed ] [ Google Scholar ]
- Tung CK, Hu L, Fiore AG, Ardon F, Hickman DG, Gilbert RO, Suarez SS and Wu M 2015b. Microgrooves and fluid flows provide preferential passageways for sperm over pathogen tritrichomonas foetus . Proceedings of the National Academy of Sciences USA 112 , 5431–5436. [ PMC free article ] [ PubMed ] [ Google Scholar ]
- Tyler KR 1977. Histological changes in the cervix of the rabbit after coitus . Journal of Reproduction and Fertility 49 , 341–345. [ PubMed ] [ Google Scholar ]
- Vishwanath R and Shannon P 2000. Storage of bovine semen in liquid and frozen state . Animal Reproduction Science 62 , 23–53. [ PubMed ] [ Google Scholar ]
- Wilmut I and Hunter RH 1984. Sperm transport into the oviducts of heifers mated early in oestrus . Reproduction Nutrition and Development 24 , 461–468. [ PubMed ] [ Google Scholar ]
- Wrobel KH, Kujat R and Fehle G 1993. The bovine tubouterine junction: General organization and surface morphology . Cell and Tissue Research 271 , 227–239. [ PubMed ] [ Google Scholar ]
- Yamaguchi R, Yamagata K, Ikawa M, Moss SB and Okabe M 2006. Aberrant distribution of adam3 in sperm from both angiotensin-converting enzyme (ace)- and calmegin (clgn)-deficient mice . Biology of Reproduction 75 , 760–766. [ PubMed ] [ Google Scholar ]
- Yamaguchi R, Muro Y, Isotani A, Tokuhiro K, Takumi K, Adham I, Ikawa M and Okabe M 2009. Disruption of adam3 impairs the migration of sperm into oviduct in mouse . Biology of Reproduction 81 , 142–146. [ PubMed ] [ Google Scholar ]
- Yang X, Zhao Y, Yang X and Kan FW 2015. Recombinant hamster oviductin is biologically active and exerts positive effects on sperm functions and sperm-oocyte binding . PLoS ONE 10 , e0123003. [ PMC free article ] [ PubMed ] [ Google Scholar ]
- Yousef MS, Marey MA, Hambruch N, Hayakawa H, Shimizu T, Hussien HA, Abdel-Razek AK, Pfarrer C and Miyamoto A 2016. Sperm binding to oviduct epithelial cells enhances tgfb1 and il10 expressions in epithelial cells as well as neutrophils in vitro: Prostaglandin e2 as a main regulator of anti-inflammatory response in the bovine oviduct . PLoS ONE 11 , e0162309. [ PMC free article ] [ PubMed ] [ Google Scholar ]

An official website of the United States government
Here’s how you know
Official websites use .gov A .gov website belongs to an official government organization in the United States.
Secure .gov websites use HTTPS A lock ( Lock Locked padlock icon ) or https:// means you’ve safely connected to the .gov website. Share sensitive information only on official, secure websites.

- Health Topics
- Drugs & Supplements
- Medical Tests
- Medical Encyclopedia
- About MedlinePlus
- Customer Support
Sperm release pathway
Sperm are produced and released by the male reproductive organs.
The testes are where sperm are produced. The testes are linked to the rest of the male reproductive organs by the vas deferens, which extends over the base of the pelvic bone or ilium, and wraps around to the ampulla, seminal vesicle, and prostate. The urethra then runs from the bladder through the penis.
Sperm production in the testes takes place in coiled structures called seminiferous tubules.
Along the top of each testicle is the epididymis. This is a cordlike structure where the sperm mature and are stored.
The release process starts when the penis fills with blood and becomes erect. Continuing to stimulate the penis will cause an ejaculation.
Mature sperm begin their journey by travelling from the epididymis to the vas deferens, which propels sperm forward with smooth muscle contractions.
The sperm arrive first at the ampulla just above the prostate gland. Here, secretions from the seminal vesicle located next to the ampulla are added.
Next, the seminal fluid is propelled forward through the ejaculatory ducts toward the urethra. As it passes the prostate gland, a milky fluid is added to make semen.
Finally, the semen is ejaculated from the penis through the urethra.
Review Date 10/15/2023
Updated by: Kelly L. Stratton, MD, FACS, Associate Professor, Department of Urology, University of Oklahoma Health Sciences Center, Oklahoma City, OK. Also reviewed by David C. Dugdale, MD, Medical Director, Brenda Conaway, Editorial Director, and the A.D.A.M. Editorial team.
Related MedlinePlus Health Topics
- Male Infertility
Thank you for visiting nature.com. You are using a browser version with limited support for CSS. To obtain the best experience, we recommend you use a more up to date browser (or turn off compatibility mode in Internet Explorer). In the meantime, to ensure continued support, we are displaying the site without styles and JavaScript.
- View all journals
- Explore content
- About the journal
- Publish with us
- Sign up for alerts
- 21 May 2024
Lab-grown sperm and eggs: ‘epigenetic’ reset in human cells paves the way
- Heidi Ledford
You can also search for this author in PubMed Google Scholar
You have full access to this article via your institution.
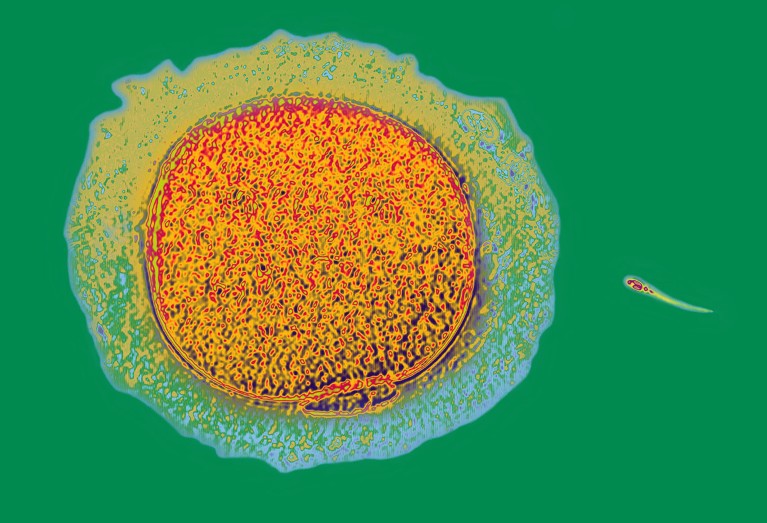
A sperm cell (right) swims towards a human egg (artificially coloured). Credit: AJ Photo/Science Photo Library
The day when human sperm and eggs can be grown in the laboratory has inched a step closer, with the discovery of a way to recreate a crucial developmental step in a dish 1 .
The advance, described on 20 May in Nature , addresses a major hurdle: how to ensure that the chemical tags on the DNA and associated proteins in artificially produced sperm and eggs are placed properly. These tags are part of a cell’s ‘ epigenome ’ and can influence whether genes are turned on or off. The epigenome changes over a person’s lifetime; during the development of the cells that will eventually give rise to sperm or eggs, these marks must be wiped clean and then reset to their original state .
“Epigenetic reprogramming is key to making the next generation,” says Mitinori Saitou, a stem-cell biologist at Kyoto University in Japan, and a co-author of the paper. He and his team worked out how to activate this reprogramming — something that had been one of the biggest challenges in generating human sperm and eggs in the laboratory, he says.
But Saitou is quick to note that there are further steps left to conquer, and that the epigenetic reprogramming his lab has achieved is not perfect.
“There is still much work to be done and considerable time required to address these challenges,” agrees Fan Guo, a reproductive epigeneticist at the Chinese Academy of Sciences Institute of Zoology in Beijing.
Eggs in a dish
Growing human sperm and eggs in the laboratory would offer hope to some couples struggling with infertility. It would also provide a way to edit disease-causing DNA sequences in sperm and eggs, sidestepping some of the technical complications of making such edits in embryos. And understanding how eggs and sperm develop can give researchers insight into some causes of infertility.
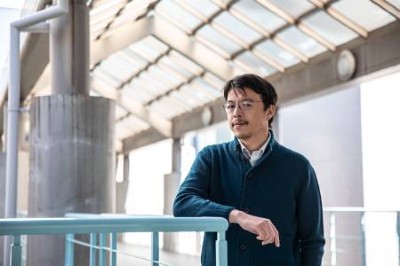
Making mice with two dads: this biologist rewrote the rules on sexual reproduction
But in addition to its technical difficulty, growing eggs and sperm in a dish — called in vitro gametogenesis — would carry weighty social and ethical questions . Genetic modification to prevent diseases, for example, could lead to genetic enhancement to boost traits associated with intelligence or athleticism.
Epigenetic reprogramming is key to the formation of reproductive cells — without it, the primordial cells that would eventually give rise to sperm and eggs stop developing. Furthermore, the epigenome affects gene activity , helping cells with identical DNA sequences to take on unique identities. The epigenome helps to differentiate a brain cell, for example, from a liver cell.
Researchers know how to grow mouse eggs and sperm using stem-cell-like cells generated from skin . But the protocols used don’t work in human cells: “There is a big gap between mice and humans,” says Saitou.
Pressing reset on the epigenome
So Saitou and his colleagues began an arduous search for a way to control epigenetic reprogramming in human cells. They found that a protein called BMP2 was essential for this step and that adding it to their cultures promoted epigenetic reprogramming. The cells grown in this culture were able to progress a step further in their development than were cells in cultures without added BMP2.
After epigenetic reprogramming, the cells’ development halted again. Even so, each step towards in vitro gametogenesis holds “immense significance”, says Guo. Saitou and his colleagues are now hunting for ways to nudge the cells further along the path to becoming sperm and eggs.
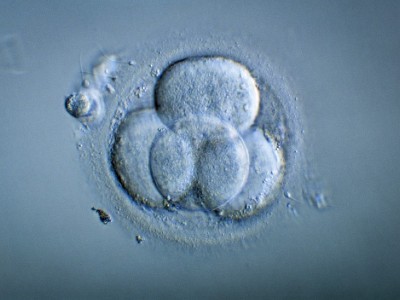
Embryos with DNA from three people develop normally in first safety study
The researchers carefully analysed epigenetic marks in their laboratory-grown cells and found that although many of these imprints had been wiped away, a few remained. This means that the reprogramming might be incomplete — which could have serious consequences if such cells were used for reproduction. “If imprinting on even one gene is aberrant, that could lead to disease,” says Saitou.
Such caveats are important to bear in mind, he says: the field of in vitro gametogenesis is advancing rapidly, and these results, along with other developments in the past few years , could fuel speculation and false claims that a solution is just around the corner. “I think in maybe five years or so, things will get more settled,” he says. “And then only the good science will remain.”
Nature 629 , 984 (2024)
doi: https://doi.org/10.1038/d41586-024-01404-x
Murase, Y. et al. Nature https://doi.org/10.1038/s41586-024-07526-6 (2024).
Article Google Scholar
Download references
Reprints and permissions
Related Articles
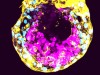
- Epigenetics
- Medical research
- Developmental biology
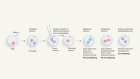
The phenomenon of genomic imprinting was discovered 40 years ago
News & Views 14 MAY 24
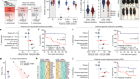
Paternal microbiome perturbations impact offspring fitness
Article 01 MAY 24
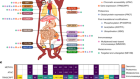
Temporal dynamics of the multi-omic response to endurance exercise training
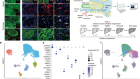
Acquisition of epithelial plasticity in human chronic liver disease
Article 22 MAY 24
In vitro reconstitution of epigenetic reprogramming in the human germ line
Article 20 MAY 24
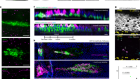
Airway hillocks are injury-resistant reservoirs of unique plastic stem cells
Don’t leave out joints and bones in exercise studies
Correspondence 28 MAY 24
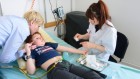
The immune system can sabotage gene therapies — can scientists rein it in?
News 28 MAY 24
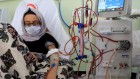
Ozempic keeps wowing: trial data show benefits for kidney disease
News 24 MAY 24
Postdoctoral Associate- Neuroscience
Houston, Texas (US)
Baylor College of Medicine (BCM)
Call for applications- junior and senior scientists
The BORDEAUX INSTITUTE OF ONCOLOGY (BRIC U1312, https://www.bricbordeaux.com/) is seeking to recruit new junior and senior researchers
Bordeaux (Ville), Gironde (FR)
INSERM - U1312 BRIC
Postdoctoral Scholar - Organic Synthesis
Memphis, Tennessee
The University of Tennessee Health Science Center (UTHSC)
Postdoctoral Scholar - Chemical Biology
Postdoctoral scholar - clinical pharmacy & translational science.
Sign up for the Nature Briefing newsletter — what matters in science, free to your inbox daily.
Quick links
- Explore articles by subject
- Guide to authors
- Editorial policies

- Consulting Editors
- For authors
- Publication ethics
- Publication alerts by email
- Advertising
Advertisement
Review Series Free access | 10.1172/JCI41585
Fertilization: a sperm’s journey to and interaction with the oocyte
Masahito ikawa, 1 naokazu inoue, 1 adam m. benham, 1,2 and masaru okabe 1.
1 Research Institute for Microbial Diseases, Osaka University, Osaka, Japan. 2 School of Biological and Biomedical Sciences, Durham University, United Kingdom.
Address correspondence to: Masaru Okabe, Research Institute for Microbial Diseases, Osaka University, Yamadaoka 3-1, Suita, Osaka, 565-0871, Japan. Phone: 81.6.6879.8338; Fax: 81.6.6879.8376; E-mail: [email protected] .
Find articles by Ikawa, M. in: JCI | PubMed | Google Scholar
Find articles by Inoue, N. in: JCI | PubMed | Google Scholar
Find articles by Benham, A. in: JCI | PubMed | Google Scholar
Find articles by Okabe, M. in: JCI | PubMed | Google Scholar
Published April 1, 2010 - More info

Mammalian fertilization comprises sperm migration through the female reproductive tract, biochemical and morphological changes to sperm, and sperm-egg interaction in the oviduct. Recent gene knockout approaches in mice have revealed that many factors previously considered important for fertilization are largely dispensable, or if they are essential, they have an unexpected function. These results indicate that what has been observed in in vitro fertilization (IVF) differs significantly from what occurs during “physiological” fertilization. This Review focuses on the advantages of studying fertilization using gene-manipulated animals and highlights an emerging molecular mechanism of mammalian fertilization.
In the early 1950s, Min Chueh Chang and Colin Russell Austin independently found that mammalian sperm must spend some time in the female reproductive tract before they acquire the ability to fertilize eggs ( 1 , 2 ). The phenomenon underlying the acquisition of fertilization capability is called sperm capacitation ( 3 ), and the discovery of Chang and Austin made it possible to perform mammalian fertilization in vitro by mixing capacitated sperm with ovulated eggs, a procedure used by in vitro fertilization (IVF) clinics today ( 4 ) (Figure 1 ). This simplified in vitro experimental system ( 5 ) also allowed researchers to intensively study the mechanism of fertilization. Indeed, using this system together with biochemical approaches, such as competitive binding of antibodies and ligands that interact with sperm, eggs, and their surroundings, various factors were reported to be important for mammalian fertilization.
Mechanism of sperm-egg interaction. Over the nucleus of each mammalian sperm is a membranous sac known as the acrosome, which is filled with many kinds of hydrolytic enzymes. In the female reproductive tract or in an IVF medium, sperm undergo capacitation, which permits the acrosome reaction. Near the eggs, probably stimulated by the cumulus cells and the ZP, sperm release their acrosomal contents by exocytosis and penetrate the ZP. Only acrosome-reacted sperm fuse with eggs, but their competency for fusion does not last long. Cumulus cells are packed together by hyaluronic acid at ovulation and become diffuse during fertilization. PVS, perivitelline space.
The development of gene-knockout technology ( 6 , 7 ) has allowed researchers to test in vivo the findings from IVF studies. Numerous factors considered to be key molecules have been examined for their importance during fertilization (Tables 1 and 2 ). Surprisingly, these experiments revealed that many of the sperm factors thought to be important for fertilization, including the acrosomal protease acrosin (Acr) ( 8 ), the zona pellucida (ZP) binding protein β-1,4-galactosyltransferase 1 (B4galt1) ( 9 , 10 ), and the egg fusion protein fertilin (a heterodimer that consists of two subunits, a disintegrin and metallopeptidase domain 1b [Adam1b] and Adam2) ( 11 ), were not essential. It can be argued that in vivo, compensatory mechanisms supplant the function of the gene targeted in a knockout mouse. However, these factors were identified as important for fertilization in studies using antibodies that blocked IVF by targeting a single epitope of these factors, and no compensatory mechanisms were detected. Although the likelihood is low that compensatory mechanisms are revealed in gene-knockout mice, it can still be argued that in knockout animals upregulation of unknown, functionally related molecules could take place. Despite these arguments, the view that genes essential for mammalian fertilization do exist has been confirmed by the discovery of proteins whose gene disruptions prevent fertility both in vitro and in vivo (e.g., cation channel, sperm associated 1 [Catsper1], ref. 12 , and izumo sperm-egg fusion 1 [Izumo1], ref. 13 ). An additional benefit of gene-knockout studies is that they reveal factors that would never have emerged as essential for fertilization from IVF experiments (e.g., angiotensin-converting enzyme [Ace], ref. 14 ; calmegin [Clgn], ref. 15 ; and Cd9, refs. 16 – 18 ). In this Review, we discuss the current understanding of the mechanism of mammalian fertilization, which has been defined mainly through in vivo analysis of various gene-manipulated animals.
Genes related to fertility that have been knocked out in mice (A to O)
Genes related to fertility that have been knocked out in mice (P to Z)
Capacitation is the process by which sperm become competent to fertilize an egg (Figure 1 ). Capacitation takes place after ejaculation, in the female reproductive tract, and is required only by mammalian sperm. Cholesterol is particularly abundant in seminal plasma ( 19 ) and has an inhibitory effect on sperm capacitation. During capacitation, cholesterol and other sterols are removed from the sperm surface, and non-covalently attached glycoproteins acquired in the epididymis are released from the sperm surface ( 20 ). Together, these modifications create a more fluid membrane environment, making the sperm competent for subsequent fertilization cues.
There are many papers demonstrating the importance of protein phosphorylation, particularly tyrosine phosphorylation, and calcium ion influx for capacitation. These signaling events may also be important for the release of putative “decapacitation factor(s)” from sperm ( 21 ). In the IVF clinic, sperm are prepared by the “swim up” method and/or by Percoll density gradient centrifugation to ensure that the sperm are free from seminal plasma, which is believed to contain decapacitation factor(s). Since individual sperm change their biochemical status after collection and incubation and there is no good method to separate them, it is inevitable that in the laboratory, mixed populations of millions of gametes are analyzed at different stages of capacitation and/or the acrosome reaction. It is important to be aware that the global biochemical status of many unsuccessful sperm may not necessarily reflect the molecular events that occur in the one successful sperm that navigates the oviduct and penetrates the ZP, the transparent glycoprotein extracellular matrix that surrounds the egg (Figure 1 ).
Mammalian sperm have to travel a long distance through the female reproductive tract to the oviduct, in which fertilization takes place. To accomplish the journey to the egg, sperm are equipped to overcome various obstacles that lie ahead, such as navigating the uterotubal junction (UTJ) and penetrating the egg extracellular matrices. For example, the cysteine-rich cationic polypeptide defensin β 126 (DEFB126) has been suggested to coat sperm, and its overall negative charge facilitates sperm attachment to the oviduct epithelium, promoting penetration of the cervical mucus ( 22 ). While the function of DEFB126, and other related epididymis-specific defensins, remains to be fully tested in knockout animals, it seems that proteins secreted from male accessory glands regulate sperm migration.
The oviduct is comprised of three major segments: the UTJ, the isthmus, and the ampulla (Figure 2 ). These structures seem to play distinct roles in ensuring successful fertilization. The UTJ connects the uterus and oviduct by intersecting the distal part of the oviduct. Several lines of evidence obtained from knockout mice suggest that sperm migration through the oviduct is biologically regulated. The sperm from male mice lacking one of the molecules Clgn, Ace, Adam1a, Adam2, or Adam3 are motile but not able to pass through the UTJ ( 23 – 27 ). For Ace, it is the testicular isoform of the protein, which is transcribed from testis-specific promoters in intron 12, that is required for fertility. Adam1a, Adam2, and Adam3 are all glycoproteins with EGF-like and peptidase M12B domains. Clgn is a testis-specific homolog of the ER-resident lectin-like chaperone calnexin. Proteomic analysis of sperm from the mutant mouse lines revealed that the sperm surface protein Adam3 is commonly absent or dislocated into detergent-rich membrane domains in all five lines of mutant mice ( 28 ). Initially, Adam3 –/– mice were reported to have no defect in sperm migration ( 29 ), but using fluorescent protein–tagged transgenic sperm and live imaging technology (Figure 2 ), a defect in the oviductal migration of Adam3 –/– sperm was revealed ( 27 ). Adam3 is now considered to be the most important factor in sperm migration in the mouse. The precise mechanism of how Adam3 facilitates the passage of sperm through the UTJ is unknown. The relationship between the five molecules that cause the disappearance of Adam3 from sperm will be discussed later in the section on sperm-ZP binding.
Sperm migration through the female reproductive tract. After sperm are deposited in the female reproductive environment, they become metabolically active and start migrating into the oviduct. ( A and B ) Depicted here is one oviduct in a female mouse. The generation of sperm engineered to express fluorescent proteins has facilitated visualization of sperm migration through the female reproductive tract ( 65 , 66 ). ( C ) Sperm from mice engineered to express acrosome-targeted EGFP (acrEGFP) and mitochondria-targeted red fluorescent protein (mtDsRed2). GFP is released during the acrosome reaction, so that acrosome-reacted sperm are no longer acrEGFP + but remain mtDsRed2 + ( 66 ). The mice are available through public bioresource centers. ( B and D – F ) The female mouse reproductive tract removed at four hours after coitus. The sperm and their acrosomal status can be monitored through the uterine and oviduct wall. Areas indicated in D and E are merged in F . The images in B , D , and E are a composite of several images. Scale bar: 500 μm ( B , D , and E ). Am, ampulla; Is, isthmus.
After passing through the UTJ, sperm are held on the surface of mucosal folds in the isthmus and remain there until the time of ovulation draws near. In cows, binder of sperm protein (BSP) on the sperm surface and annexin family proteins on the oviduct epithelial surface have been suggested to play important roles in sperm storage in the isthmus ( 30 , 31 ). BSPs are fibronectin type II domain–containing proteins released from the male seminal vesicles (a pair of simple tubular glands that release their contents into the vas deferens), and include PDC109 (BSPA1/A2), BSPA3, and BSP30K. Recently, BSP homologs were found in the human (BSPH1) and mouse ( 32 ). It has also been suggested that the reducing redox environment of the oviduct could promote the release of sperm by facilitating the reduction of cell surface thiols on sperm cell proteins important for sperm-oviduct binding ( 33 ).
During sperm storage, the isthmic epithelium creates a microenvironment that delays capacitation and stabilizes sperm for a period of approximately 24 hours, at least in humans ( 34 , 35 ). When ovulation draws near, unknown female factors trigger the sperm to leave the reservoir and move up to the ampulla. Release of sperm from the isthmic epithelium is reported to depend mainly on sperm changes that are associated with capacitation. For example, bull sperm are reported to shed their BSPs and lose their oviduct epithelium–binding ability ( 36 ). Hyperactivated sperm movement and asymmetrical beating of the sperm flagellum occurs after capacitation and is thought to assist sperm escaping from the oviduct epithelium ( 37 ). The importance of hyperactivated sperm movement in release from the isthmic epithelium is supported by a report that Catsper1 –/– sperm are unable to detach from oviduct epithelium ( 38 ). A functional CATSPER channel has been reported to control calcium entry, which stimulates the hyperactivated state ( 12 , 39 , 40 ). Of clinical interest, human male infertility caused by mutations in the gene encoding CATSPER1 was reported recently ( 41 ).
The gradual release of sperm from the isthmus helps to reduce the number of sperm available at the point of fertilization and avoids polyspermy (fertilization of an egg by more than one sperm), which is fatal for embryonic development ( 42 ). Surgical removal of the isthmus in the pig leads to increased numbers of sperm entering the ampulla and polyspermy in about one-third of eggs ( 43 ), suggesting that the isthmic portion of the female reproductive tract regulates the number of fertilization-competent sperm that reach the egg in vivo. This control step is bypassed by IVF, which usually requires thousands of sperm to occur successfully.
After leaving the storage reservoir, sperm move into the ampulla and locate the cumulus-cell oocyte complex (COC). The COC is comprised of ovulated eggs covered by the ZP and a multicellular cumulus oophorus. Sperm chemotaxis is implicated in locating the COC. In particular, human sperm have been reported to sense a chemoattractant from both follicular fluid ( 44 ) and COCs ( 45 ). Progesterone was proven to be the cumulus-derived chemoattractant by the observation that antiprogesterone treatment abrogated the in vitro chemotactic activity of human ( 46 ) and rabbit ( 47 ) cumulus-cultured medium. Olfactory receptor, family 1, subfamily D, member 2 (OR1D2) was also demonstrated to function in human sperm chemotaxis and induced calcium signaling when sperm were exposed in vitro to the representative chemical attractant bourgeonal (an aromatic aldehyde) ( 48 ). The contribution of these factors to in vivo fertilization awaits analysis by gene-knockout approaches.
Soon after interacting with COCs, sperm penetrate the matrix of the cumulus oophorus, which is rich in proteins and carbohydrates such as hyaluronan, an unsulfated glycosaminoglycan (Figure 1 ). In marsupials, eggs shed their cumulus layers just before ovulation, and the cumulus-free eggs are fertilized in vivo ( 49 , 50 ). Mouse eggs freed from cumulus using hyaluronidase can be fertilized in vitro. However, the cumulus layers surrounding the mouse oocyte are beneficial for fertilization, and genetic deletion of a number of genes involved in synthesizing and stabilizing the COC extracellular matrix suppresses fertilization in vivo. Female mice lacking any one of the factors α1 microglobulin/bikunin (Ambp) ( 51 ), TNF-α–induced protein 6 (Tnfip6) ( 52 , 53 ), and pentraxin 3 (Ptx3) ( 54 ) have been shown to exhibit reduced female fertility, due to defects in the integrity of the COC. In these female mice, the number of ovulated oocytes in the oviduct was reduced, but the oocytes that reached the oviduct showed impaired fertilizing ability.
It was recently reported that cumulus cells secrete CCL chemokines upon activation of the TLR system by hyaluronan fragments generated by sperm hyaluronidase ( 55 ). The study of prostaglandin E receptor subtype EP2–knockout ( Ptger2 -knockout) mice also supports the notion that CCL chemokines facilitate sperm migration to the COC. Ptger2 is expressed in cumulus cells and helps to lower the release of Ccl7. Without Ptger2 , release of Ccl7 is increased and prevents fertilization by causing the cumulus extracellular matrix to harden ( 56 ). Timely interaction between prostaglandin and chemokine signaling in the cumulus may assist monospermic fertilization.
Sperm adhesion molecule 1 (Spam1) was first identified as a sperm receptor for the ZP but was later proven to have hyaluronidase activity and was implicated in sperm passage through the COC ( 57 , 58 ). The originally described ZP-binding ability is not physiologically essential, because Spam1 –/– sperm have been shown to bind the ZP. Spam1 -disrupted mice are fertile, although the mutant sperm show a reduced ability to disperse cumulus cells in vitro ( 59 ). Tadashi Baba and colleagues found that Spam1 –/– sperm retain approximately 40% of their hyaluronidase activity and identified hyaluronoglucosaminidase 5 (Hyal5) as an additional sperm-specific hyaluronidase ( 60 ). When they generated Hyal5 –/– mice, sperm fertility was comparable to that of wild-type mice, both in vitro and in vivo ( 61 ), suggesting a more important role for Spam1 than Hyal5 during sperm passage through the cumulus layers. It will be interesting to determine the details of the sperm fertilizing ability in Spam1 –/– Hyal5 –/– double-knockout mice in the future.
The acrosome is a Golgi-derived exocytotic organelle that covers the tip of the sperm head (Figure 1 ). Acrosomal exocytosis, the so-called acrosome reaction, happens only in capacitated sperm and is a prerequisite for a sperm to fuse with an egg (Figure 1 ). Because of its physiological importance, various methods have been proposed to assess acrosomal status ( 62 – 64 ). Taking advantage of mice engineered to express GFP in the acrosome, we have been able to observe, in real-time, the acrosome reaction in live sperm without any pretreatment (Figure 2 ) ( 65 , 66 ). It is noteworthy that the acrosome reaction is not a simple all-or-none event but one with intermediate stages. While the soluble GFP disperses from the acrosome within seconds ( 65 ), other acrosomal components are only gradually released. For example, the MN7 and MC41 (acrin1 and acrin2) acrosomal antigens remain attached to the sperm head for at least 15 minutes ( 67 ). Further investigation of these intermediate stages of fertilization is awaited ( 68 ).
The acrosome reaction can be induced in vitro with solubilized ZP ( 69 – 71 ). However, there is a report showing that the intact ZP is not sufficient to induce acrosomal exocytosis ( 72 ). Further, according to Ryuzo Yanagimachi, some mouse sperm passing through cumulus layers are already acrosome reacting and have reacted before reaching the ZP ( 73 ). In shrews, the acrosome reaction is induced by cumulus cells but not the ZP ( 74 ). Progesterone secreted from human cumulus cells is reported to induce the acrosome reaction ( 75 ). Moreover, 20%–40% of capacitated mouse sperm spontaneously undergo the acrosome reaction in an IVF medium, such as TYH ( 76 ), without any natural inducer, and those sperm are efficiently able to fertilize in vitro eggs without the cumulus oophorus and ZP. Therefore, a direct sperm-ZP interaction does not seem to be necessary for the acrosome reaction and thus may not be “essential” for fertilization, even in vivo (Figure 1 ).
The mechanism of the acrosome reaction itself has been well characterized. In brief, transient calcium influx leads to activation of phospholipase C (PLC), and activated PLC generates IP3 and diacylglycerol (DAG) from PIP2. IP3 releases calcium from intracellular stores, and DAG mediates PKC activation and phosphorylation of substrate proteins. These early events promote a subsequent calcium influx via transient receptor potential cation channels (TRPCs), which induces the complete acrosome reaction. Disruption of Plcd4 impairs the in vitro ZP-induced acrosome reaction, while the A23187 ionophore-induced acrosome reaction occurs normally ( 71 ).
There is growing evidence that the soluble NSF attachment protein receptor (SNARE) complex regulates the sperm acrosome reaction. Sperm from hydrocephaly with hop gait (Hyh) mice, which have a spontaneous point mutation in the α-SNAP–encoding gene ( Napa ), exhibit severely impaired fertility ( 77 ). The problem was traced to a defect in the ZP-induced acrosome reaction. Mice lacking complexin-I (Cplx1), which associates with the SNARE complex and modulates its function ( 78 ), also generate sperm with an impaired ability to undergo progesterone-induced acrosome reactions ( 79 ). It should be noted that although Plcd4 –/– , Hyh, and Cplx1 –/– mutant sperm are severely impaired in their ability to undergo ligand-induced acrosome reactions, these sperm are still able to fertilize 56.9%, 46.3%, and 47.3% of eggs, respectively, in vitro. These data support the idea that the ligand-induced acrosome reaction is not essential but assists fertilization.
After passing through the cumulus oophorus, sperm encounter the ZP, their last hurdle before meeting the egg (Figure 1 ). The major components of the ZP are three glycosylated proteins, Zp1, Zp2, and Zp3. There are various reports indicating that Zp3 functions as the primary sperm receptor and can induce the acrosome reaction ( 80 – 82 ). During the passage of sperm through the ZP, Zp2 is thought to function as a secondary receptor for acrosome-reacted sperm. On fertilized eggs, Zp2 is converted to Zp2f by an oocyte secretory enzyme(s) to prevent further sperm binding and fertilizing the egg ( 83 , 84 ). Zp1 is thought to cross-link Zp2/Zp3 heterodimers and creates the filamentous structure of the ZP ( 85 ). Interestingly, knockout studies have revealed that eggs can form the ZP in the absence of either Zp1 or Zp2 and that sperm can fertilize these eggs ( 86 , 87 ). However, when Zp3 was disrupted, the ZP was not formed ( 88 , 89 ). Although oocytes lacking a ZP or with only a thin ZP ( Zp3 –/– and Zp2 –/– oocytes, respectively) were able to be fertilized with wild-type sperm, their developmental ability was compromised ( 87 ). These results indicate that the ZP helps to maintain an appropriate interaction between granulose cells and oocytes during oocyte maturation. ZP4 has been identified in some species, including humans, but its species-specific function(s) remains to be determined ( 90 ).
The ZP not only functions as a receptor for sperm but also acts as a species-specific barrier ( 73 ). Rankin et al. replaced mouse Zp2 and Zp3 with their human homologs and examined the fertilization potential of eggs surrounded with a mouse Zp1/human ZP2/human ZP3 chimeric ZP ( 91 ). Mouse sperm, but not human sperm, were able to bind to the chimeric ZP and fertilize the eggs. As Zp3 is thought to be the primary sperm receptor, these data suggest that oligosaccharides attached to the ZP proteins, rather than the peptide sequences themselves, are critical for species-specific sperm binding. This idea is also supported by earlier biochemical studies, showing that enzymatic removal of terminal galactose (Gal) or N-acetylglucosamine (GlcNac) residues from the ZP abolishes its affinity for sperm ( 92 – 94 ). However, it has been reported that disruption of mannoside acetylglucosaminyltransferase 1 (Mgat1), a medial-Golgi enzyme essential for the synthesis of hybrid and complex N-glycans, resulted in oocytes that were efficiently fertilized, even though the ZP was fragile and lacked terminal N-glycan Gal and GlcNac residues ( 95 ). The same group also disrupted T-synthase (C1galt1) so that ZP without core 1 and core 2 O-glycans was generated. Since there are no core 3 and core 4 O-glycans in the ZP, these mice can be considered as having O-glycan–deficient ZP. However, oocytes from these animals were still fertilized by sperm ( 96 ). Moreover, oocyte-specific disruption of both Mgat1 and C1galt1 has been achieved, and ZP with no terminal Gal and GlcNac was shown to be functional ( 96 ). The results obtained from gene-knockout studies using oocyte-specific Cre recombinase strongly support the idea that the oligosaccharides on the ZP are far less important for sperm-egg interactions than previously believed. An alternative interpretation could be that there are some unknown ZP-associated glycoproteins (derived from ovary or another source where the glycosyl transferases are still expressed) that are important for sperm-egg interactions.
Various reports exist supporting the notion that B4galt1 on the sperm surface can bind to ZP glycans ( 97 ). However, when B4galt1 -knockout mice were produced, the mutant sperm could fertilize eggs ( 9 , 10 ). The literature is replete with examples of sperm factors that have been demonstrated to be important for ZP binding, using a biochemical approach, but later proven to be not essential in gene-disruption experiments.
Ironically, the first case of normal-looking sperm with defective ZP-binding capacity was generated unexpectedly ( 15 ). When sperm from Clgn –/– mice were mixed with cumulus-free eggs, the sperm were unable to bind the ZP and were repelled. However, Clgn is not directly involved in sperm-egg interactions, because Clgn is a testis-specific ER molecular chaperone involved in folding newly synthesized secretory and membrane proteins. Even in wild-type male mice, Clgn is absent from mature sperm. We therefore speculated that Clgn facilitates the maturation of a sperm protein(s) required for ZP binding.
Five knockout mouse strains have now been reported to show defective sperm-ZP binding ( Clgn -, Ace -, Adam1a -, Adam2 -, and Adam3 -knockout mice) (Figure 3 ). The disruption of Clgn impairs Adam1a/Adam2 and Adam1b/Adam2 heterodimerization, and because these other ADAM family members regulate the amount of Adam3 on sperm, this leads to the complete loss of Adam2 and Adam3 from the surface of mature sperm ( 23 , 28 ). When Baba and colleagues produced Adam1b -knockout mice, Adam2 was also found to disappear from sperm, but Adam3 remained intact and the sperm were fertile ( 11 ). When Adam1a , which is ER specific and not found in mature sperm, was knocked out, Adam2 remained intact, but the sperm lost surface expression of Adam3 and ZP-binding ability ( 25 ). This result clearly indicates that Adam1b/Adam2 (i.e., fertilin) is dispensable for fertilization, at least in mice. This result is surprising, because there was strong evidence that the disintegrin domain of fertilin on sperm interacted with the RGD domain of integrins on oocytes and that the addition of RGD fragments to sperm inhibited fertilization. One could argue that some other factor(s) compensates for the loss of fertilin in gene-disrupted mice during spermatogenesis, but that the same factor(s) is not able to compensate for the loss of fertilin function when inhibitory antibodies or exogenous ligands are added. However, it could be counter-argued that the fertilization inhibiting activity of the antibody and/or ligands was not physiological but rather an artifactual effect. Since the entire mechanism of fertilization is yet to be clarified, the outcome of the debate awaits further investigations.
Maturation of ADAMs and their roles in sperm function. Disruption of the genes Clgn , Ace , Adam1a , Adam2 , and Adam3 results in impaired sperm-ZP binding and impaired migration through the UTJ. Clgn is required for Adam1a/Adam2 and Adam1b/Adam2 heterodimerization. Lack of Adam1a/Adam2 heterodimerization in Clgn –/– , Adam1a –/– , and Adam2 –/– mice causes Adam3 disappearance from the surface of mature sperm. Disruption of Ace leads to aberrant localization of Adam3, as evidenced by reduced amounts of Adam3 protein in the Triton X-114 detergent-enriched phase of sperm membranes ( 28 ). The diagram illustrates why disruption of the individual Ace , Clgn , Adam1a , Adam2 , and Adam3 genes produces similar phenotypes and indicates the importance of Adam3 in sperm fertilizing ability.
The Adam3 protein can directly bind to the ZP ( 60 ). It appears that Adam3 requires the concerted action of Clgn and Adam1a/Adam2 for its quality control and maturation during spermatogenesis. The mechanism of infertility caused by Ace disruption remained unclear for many years, since overall levels of ADAMs in sperm are not influenced by Ace. Recently, however, we noticed a substantial decrease in the amount of Adam3 in membrane microdomains and suggested that erroneous distribution of Adam3 in Ace –/– mice might cause the defective ZP-binding phenotype ( 28 ) (Figure 3 ). These observations point toward Adam3 as the most important factor that participates in sperm-ZP binding. However, in humans, other ADAMs, or another protein, are likely to function as an alternative to Adam3, because the human homolog of Adam3 seems to be a pseudo gene ( 98 , 99 ). In fact, there are various papers showing that other proteins are involved in human sperm binding to the ZP, including the 56-kDa Zp3 receptor (ZP3R) and zonadhesin (ZAN). Zp3r is a peripheral membrane glycoprotein that has been suggested to be a receptor for Zp3 in the mouse ( 100 , 101 ), whereas ZAN is a large sperm head protein, with multiple isoforms, that has been implicated in species-specific gamete recognition ( 102 ).
In addition to their inability to bind to the ZP, sperm from Clgn -, Ace -, Adam1a -, Adam2 -, and Adam3 -knockout mice share another notable phenotype: they are unable to migrate into the oviduct ( 23 – 27 ). This suggests that oviduct migration and ZP binding might share a common mechanism. This hypothesis is supported by the phenotype of a sixth mutant mouse, the post-GPI attachment to proteins 1–knockout ( Pgap1 -knockout) mouse. Pgap1 is an ER resident GPI inositol-deacylase that is involved in the maturation of GPI-linked proteins. Few Pgap1 –/– mice reach sexual maturity. Those male mice that do reach adulthood have sperm that are normal in number and motility but that cannot ascend the oviduct or bind to the ZP ( 103 ). If the reason underlying this commonly observed dual defect is clarified, it will help us to understand the molecular mechanisms of fertilization in more detail.
The enzymatic hypothesis for ZP penetration posits that proteolytic cleavage of ZP proteins by sperm cell–surface proteases clears a path for the incoming sperm ( 73 ). The enzyme heralded as the prime candidate for the controlled proteolytic clearance of ZP proteins was Acr. Acr is an acrosomal enzyme with chymotryptic activity that is released during the acrosome reaction. Despite several papers supporting an important role for Acr in ZP binding and penetration, Acr –/– sperm can still fertilize eggs, albeit with a slight delay compared with wild-type sperm ( 8 , 104 ). Baba and colleagues found that protease activity persists in the sperm of Acr -knockout mice, suggesting that alternative proteases could be involved in ZP penetration. Five more testis-specific serine proteases have been identified and named Tesp1–Tesp5 ( 105 ). Thus far, only mice lacking Tesp5 (also known as Prss21) have been generated and analyzed ( 106 ). Sperm from these mice have a diminished ability to bind to the ZP and fuse with eggs, but curiously, the phenotype is rescued by treating the sperm with uterine fluids. This observation further demonstrates the complex interplay between male and female factors during the course of fertilization.
After penetration of the ZP, sperm immediately meet and fuse with the egg plasma membrane (Figure 4 ). Electron microscopic observation has shown that sperm that penetrate the ZP are acrosome reacted ( 107 , 108 ). The fact that only acrosome-reacted sperm are able to fuse with eggs implies that a sperm fusogen(s) is hidden or latent in fresh sperm and becomes exposed or activated only after the acrosome reaction. Many sperm antigens, such as Mn9, Cd46, and Izumo1, become reactive to antibodies only after the acrosome reaction has been completed. We previously raised a monoclonal antibody, OBF13, that inhibited sperm-egg fusion. The cognate antigen was a good candidate for a fusion factor ( 109 ). We identified the antigen as an Ig superfamily, type I membrane protein, with an extracellular Ig domain that contains one putative glycosylation site. The antigen was named “IZUMO” after a Japanese Shinto shrine dedicated to marriage. Izumo1 –/– male mice are completely sterile, even though the mutant sperm can penetrate the ZP and contact the egg plasma membrane ( 13 ). When the fusion step was bypassed by intracytoplasmic sperm injection into unfertilized eggs, Izumo1 –/– sperm activated eggs, and the fertilized eggs developed to term normally when transferred to the uterus of female mice. Therefore, the Izumo1 protein is essential for sperm-egg fusion ( 13 ). During the acrosome reaction, Izumo1 relocates from the anterior head of the sperm to the site(s) in which fusion will take place (Figure 4 , A–D). Testis-specific serine kinase 6 (Tssk6) is a male germ cell–specific serine kinase, and sperm from mice lacking Tssk6 have defects in ZP binding and do not redistribute Izumo1 properly ( 110 , 111 ).
Potential mechanism of sperm-egg fusion. Sperm Izumo1 and egg Cd9 are essential factors for sperm-egg fusion ( 13 , 16 ). GPI-anchored proteins on the egg surface are also essential ( 114 ), but none of the individual proteins have been identified. ( A ) Izumo1 is an acrosomal membrane protein that is not exposed before the acrosome reaction is complete. Acrosome-reacted sperm can be classified into three major groups by their Izumo1 staining pattern: acrosomal cap ( B ), equatorial ( C ), and whole head ( D ). ( E and F ) Cd9 is localized on the cilia distributed across the surface of unfertilized eggs, except the cilia at the metaphase plate. ( G ) Cd9-containing vesicles (asterisks) secreted from the oocyte are able to translocate onto the sperm surface and may play a role in sperm-egg fusion. G is reproduced with permission from Proceedings of the National Academy of Sciences of the United States of America ( 115 ).
What is essential on the egg surface? When fertilin was believed to be the essential sperm factor, α 6 β 1 integrin on the egg surface was considered a likely candidate binding partner ( 112 ). However, gene disruption experiments showed that neither α 6 integrin nor β 1 integrin were essential ( 113 ). Instead, a chance discovery led to the identification of the tetraspanin Cd9 as an egg cell–surface protein essential for fertility ( 16 – 18 ) (Figure 4 ). Cd9 is ubiquitously expressed and was expected to function in various cells. However, the Cd9 -knockout mouse showed a defect restricted to eggs, in which the protein was found to be essential for sperm-egg fusion. Given that Izumo1 is essential for sperm to bind to eggs and that Cd9 is essential for eggs to bind to sperm, it is tempting to speculate that they interact with each other to form a fusogenic complex. However, we, and others, have not detected any direct interaction between sperm Izumo1 and egg Cd9. If these proteins do indeed interact, it is likely that they both require associating proteins on the sperm and egg cell surface, and the identity of these putative factors is being intensively investigated.
Experiments using gene-manipulated animals are very powerful tools for judging which factors are essential in fertilization. Of course, if a certain factor is judged as “not essential,” it does not necessarily mean the factor does not function at all in vivo. The number of genes that are indispensable for fertilization is growing, and their roles and relationships in sperm-ZP interactions are becoming clearer. Gene-disruption experiments are conducted in many research fields, and the number of genes disrupted is increasing day by day. Thus, genes that affect reproduction will continue to be found, even by researchers in different fields. The analysis of both expected and serendipitous fertility phenotypes is steadily bringing into focus a clear image of sperm-egg interaction mechanisms. We therefore believe that the day that we can portray the sequential events in fertilization is drawing closer
We thank our colleagues and collaborators, Kenji Miyado, Yuhkoh Satouh, and Hidetoshi Hasuwa, and Yuko Muro for preparing figures. This study was partly supported by MEXT of Japan and Japan Society for the Promotion of Science.
Conflict of interest: The authors have declared that no conflict of interest exists.
Reference information: J Clin Invest. 2010;120(4):984–994. doi:10.1172/JCI41585.
- Chang MC. Fertilizing capacity of spermatozoa deposited into the fallopian tubes. Nature. 1951;168(4277):697–698. View this article via: PubMed Google Scholar
- Austin CR. Observations on the penetration of the sperm in the mammalian egg. Aust J Sci Res B. 1951;4(4):581–596. View this article via: PubMed Google Scholar
- Austin CR. The capacitation of the mammalian sperm. Nature. 1952;170(4321):326. View this article via: PubMed CrossRef Google Scholar
- Chang MC. Fertilization of rabbit ova in vitro. Nature. 1959;184(suppl 7):466–467. View this article via: PubMed CrossRef Google Scholar
- Yanagimachi R, Chang MC. Fertilization of hamster eggs in vitro. Nature. 1963;200:281–282. View this article via: PubMed Google Scholar
- Doetschman T, Maeda N, Smithies O. Targeted mutation of the Hprt gene in mouse embryonic stem cells. Proc Natl Acad Sci U S A. 1988;85(22):8583–8587. View this article via: PubMed CrossRef Google Scholar
- Mansour SL, Thomas KR, Capecchi MR. Disruption of the proto-oncogene int-2 in mouse embryo-derived stem cells: a general strategy for targeting mutations to non-selectable genes. Nature. 1988;336(6197):348–352. View this article via: PubMed CrossRef Google Scholar
- Baba T, Azuma S, Kashiwabara S, Toyoda Y. Sperm from mice carrying a targeted mutation of the acrosin gene can penetrate the oocyte zona pellucida and effect fertilization. J Biol Chem. 1994;269(50):31845–31849. View this article via: PubMed Google Scholar
- Lu Q, Shur BD. Sperm from beta 1,4-galactosyltransferase-null mice are refractory to ZP3-induced acrosome reactions and penetrate the zona pellucida poorly. Development. 1997;124(20):4121–4131. View this article via: PubMed Google Scholar
- Asano M, et al. Growth retardation and early death of beta-1,4-galactosyltransferase knockout mice with augmented proliferation and abnormal differentiation of epithelial cells. EMBO J. 1997;16(8):1850–1857. View this article via: PubMed CrossRef Google Scholar
- Kim E, et al. Mouse sperm lacking ADAM1b/ADAM2 fertilin can fuse with the egg plasma membrane and effect fertilization. J Biol Chem. 2006;281(9):5634–5639. View this article via: PubMed Google Scholar
- Ren D, et al. A sperm ion channel required for sperm motility and male fertility. Nature. 2001;413(6856):603–609. View this article via: PubMed Google Scholar
- Inoue N, Ikawa M, Isotani A, Okabe M. The immunoglobulin superfamily protein Izumo is required for sperm to fuse with eggs. Nature. 2005;434(7030):234–238. View this article via: PubMed Google Scholar
- Krege JH, et al. Male-female differences in fertility and blood pressure in ACE-deficient mice. Nature. 1995;375(6527):146–148. View this article via: PubMed CrossRef Google Scholar
- Ikawa M, et al. The putative chaperone calmegin is required for sperm fertility. Nature. 1997;387(6633):607–611. View this article via: PubMed Google Scholar
- Miyado K, et al. Requirement of CD9 on the egg plasma membrane for fertilization. Science. 2000;287(5451):321–324. View this article via: PubMed CrossRef Google Scholar
- Kaji K, et al. The gamete fusion process is defective in eggs of Cd9-deficient mice. Nat Genet. 2000;24(3):279–282. View this article via: PubMed CrossRef Google Scholar
- Le Naour F, Rubinstein E, Jasmin C, Prenant M, Boucheix C. Severely reduced female fertility in CD9-deficient mice. Science. 2000;287(5451):319–321. View this article via: PubMed CrossRef Google Scholar
- Eliasson R. Cholesterol in human semen. Biochem J. 1966;98(1):242–243. View this article via: PubMed Google Scholar
- Yano R, et al. Bactericidal/Permeability-Increasing Protein (BPI) is associated with the acrosome region of rodent epididymal spermatozoa [published online ahead of print September 10, 2009]. J Androl. doi:10.2164/jandrol.109.007880. View this article via: PubMed Google Scholar
- De Jonge C. Biological basis for human capacitation. Hum Reprod Update. 2005;11(3):205–214. View this article via: PubMed CrossRef Google Scholar
- Tollner TL, Yudin AI, Treece CA, Overstreet JW, Cherr GN. Macaque sperm coating protein DEFB126 facilitates sperm penetration of cervical mucus. Hum Reprod. 2008;23(11):2523–2534. View this article via: PubMed CrossRef Google Scholar
- Ikawa M, et al. Calmegin is required for fertilin alpha/beta heterodimerization and sperm fertility. Dev Biol. 2001;240(1):254–261. View this article via: PubMed CrossRef Google Scholar
- Hagaman JR, et al. Angiotensin-converting enzyme and male fertility. Proc Natl Acad Sci U S A. 1998;95(5):2552–2557. View this article via: PubMed Google Scholar
- Nishimura H, Kim E, Nakanishi T, Baba T. Possible function of the ADAM1a/ADAM2 Fertilin complex in the appearance of ADAM3 on the sperm surface. J Biol Chem. 2004;279(33):34957–34962. View this article via: PubMed CrossRef Google Scholar
- Cho C, et al. Fertilization defects in sperm from mice lacking fertilin beta. Science. 1998;281(5384):1857–1859. View this article via: PubMed Google Scholar
- Yamaguchi R, et al. Disruption of ADAM3 impairs the migration of sperm into oviduct in mouse. Biol Reprod. 2009;81(1):142–146. View this article via: PubMed CrossRef Google Scholar
- Yamaguchi R, Yamagata K, Ikawa M, Moss SB, Okabe M. Aberrant distribution of ADAM3 in sperm from both angiotensin-converting enzyme (Ace)- and calmegin (Clgn)-deficient mice. Biol Reprod. 2006;75(5):760–766. View this article via: PubMed CrossRef Google Scholar
- Shamsadin R, Adham IM, Nayernia K, Heinlein UA, Oberwinkler H, Engel W. Male mice deficient for germ-cell cyritestin are infertile. Biol Reprod. 1999;61(6):1445–1451. View this article via: PubMed CrossRef Google Scholar
- Ignotz GG, Cho MY, Suarez SS. Annexins are candidate oviductal receptors for bovine sperm surface proteins and thus may serve to hold bovine sperm in the oviductal reservoir. Biol Reprod. 2007;77(6):906–913. View this article via: PubMed CrossRef Google Scholar
- Suarez SS. Regulation of sperm storage and movement in the mammalian oviduct. Int J Dev Biol. 2008;52(5–6):455–462. View this article via: PubMed CrossRef Google Scholar
- Lefebvre J, Fan J, Chevalier S, Sullivan R, Carmona E, Manjunath P. Genomic structure and tissue-specific expression of human and mouse genes encoding homologues of the major bovine seminal plasma proteins. Mol Hum Reprod. 2007;13(1):45–53. View this article via: PubMed CrossRef Google Scholar
- Gualtieri R, Mollo V, Duma G, Talevi R. Redox control of surface protein sulphhydryls in bovine spermatozoa reversibly modulates sperm adhesion to the oviductal epithelium and capacitation. Reproduction. 2009;138(1):33–43. View this article via: PubMed CrossRef Google Scholar
- Dobrinski I, Smith TT, Suarez SS, Ball BA. Membrane contact with oviductal epithelium modulates the intracellular calcium concentration of equine spermatozoa in vitro. Biol Reprod. 1997;56(4):861–869. View this article via: PubMed CrossRef Google Scholar
- Murray SC, Smith TT. Sperm interaction with fallopian tube apical membrane enhances sperm motility and delays capacitation. Fertil Steril. 1997;68(2):351–357. View this article via: PubMed Google Scholar
- Ignotz GG, Lo MC, Perez CL, Gwathmey TM, Suarez SS. Characterization of a fucose-binding protein from bull sperm and seminal plasma that may be responsible for formation of the oviductal sperm reservoir. Biol Reprod. 2001;64(6):1806–1811. View this article via: PubMed CrossRef Google Scholar
- Demott RP, Suarez SS. Hyperactivated sperm progress in the mouse oviduct. Biol Reprod. 1992;46(5):779–785. View this article via: PubMed Google Scholar
- Ho K, Wolff CA, Suarez SS. CatSper-null mutant spermatozoa are unable to ascend beyond the oviductal reservoir. Reprod Fertil Dev. 2009;21(2):345–350. View this article via: PubMed CrossRef Google Scholar
- Carlson AE, et al. CatSper1 required for evoked Ca2+ entry and control of flagellar function in sperm. Proc Natl Acad Sci U S A. 2003;100(25):14864–14868. View this article via: PubMed CrossRef Google Scholar
- Qi H, et al. All four CatSper ion channel proteins are required for male fertility and sperm cell hyperactivated motility. Proc Natl Acad Sci U S A. 2007;104(4):1219–1223. View this article via: PubMed Google Scholar
- Avenarius MR, et al. Human male infertility caused by mutations in the CATSPER1 channel protein. Am J Hum Genet. 2009;84(4):505–510. View this article via: PubMed CrossRef Google Scholar
- Hunter RH. Ovarian control of very low sperm/egg ratios at the commencement of mammalian fertilisation to avoid polyspermy. Mol Reprod Dev. 1996;44(3):417–422. View this article via: PubMed CrossRef Google Scholar
- Hunter RH, Leglise PC. Polyspermic fertilization following tubal surgery in pigs, with particular reference to the role of the isthmus. J Reprod Fertil. 1971;24(2):233–246. View this article via: PubMed Google Scholar
- Cohen-Dayag A, Tur-Kaspa I, Dor J, Mashiach S, Eisenbach M. Sperm capacitation in humans is transient and correlates with chemotactic responsiveness to follicular factors. Proc Natl Acad Sci U S A. 1995;92(24):11039–11043. View this article via: PubMed CrossRef Google Scholar
- Sun F, et al. Human sperm chemotaxis: both the oocyte and its surrounding cumulus cells secrete sperm chemoattractants. Hum Reprod. 2005;20(3):761–767. View this article via: PubMed CrossRef Google Scholar
- Oren-Benaroya R, Orvieto R, Gakamsky A, Pinchasov M, Eisenbach M. The sperm chemoattractant secreted from human cumulus cells is progesterone. Hum Reprod. 2008;23(10):2339–2345. View this article via: PubMed CrossRef Google Scholar
- Guidobaldi HA, Teves ME, Unates DR, Anastasia A, Giojalas LC. Progesterone from the cumulus cells is the sperm chemoattractant secreted by the rabbit oocyte cumulus complex. PLoS One. 2008;3(8):e3040. View this article via: PubMed CrossRef Google Scholar
- Spehr M, et al. Identification of a testicular odorant receptor mediating human sperm chemotaxis. Science. 2003;299(5615):2054–2058. View this article via: PubMed CrossRef Google Scholar
- Rodger JC, Bedford JM. Separation of sperm pairs and sperm-egg interaction in the opossum, Didelphis virginiana. J Reprod Fertil. 1982;64(1):171–179. View this article via: PubMed Google Scholar
- Blandau RJ. Biology of eggs and implantation. In: Sex and Internal Secretions. Baltimore, MD: Williams & Wilkins; 1961;2:797–882.
- Zhuo L, et al. Defect in SHAP-hyaluronan complex causes severe female infertility. A study by inactivation of the bikunin gene in mice. J Biol Chem. 2001;276(11):7693–7696. View this article via: PubMed Google Scholar
- Fulop C, et al. Impaired cumulus mucification and female sterility in tumor necrosis factor-induced protein-6 deficient mice. Development. 2003;130(10):2253–2261. View this article via: PubMed Google Scholar
- Mukhopadhyay D, Asari A, Rugg MS, Day AJ, Fulop C. Specificity of the tumor necrosis factor-induced protein 6-mediated heavy chain transfer from inter-alpha-trypsin inhibitor to hyaluronan: implications for the assembly of the cumulus extracellular matrix. J Biol Chem. 2004;279(12):11119–11128. View this article via: PubMed CrossRef Google Scholar
- Varani S, et al. Knockout of pentraxin 3, a downstream target of growth differentiation factor-9, causes female subfertility. Mol Endocrinol. 2002;16(6):1154–1167. View this article via: PubMed CrossRef Google Scholar
- Shimada M, et al. Hyaluronan fragments generated by sperm-secreted hyaluronidase stimulate cytokine/chemokine production via the TLR2 and TLR4 pathway in cumulus cells of ovulated COCs, which may enhance fertilization. Development. 2008;135(11):2001–2011. View this article via: PubMed Google Scholar
- Tamba S, Yodoi R, Segi-Nishida E, Ichikawa A, Narumiya S, Sugimoto Y. Timely interaction between prostaglandin and chemokine signaling is a prerequisite for successful fertilization. Proc Natl Acad Sci U S A. 2008;105(38):14539–14544. View this article via: PubMed CrossRef Google Scholar
- Primakoff P, Lathrop W, Woolman L, Cowan A, Myles D. Fully effective contraception in male and female guinea pigs immunized with the sperm protein PH-20. Nature. 1988;335(6190):543–546. View this article via: PubMed CrossRef Google Scholar
- Lin Y, Mahan K, Lathrop WF, Myles DG, Primakoff P. A hyaluronidase activity of the sperm plasma membrane protein PH-20 enables sperm to penetrate the cumulus cell layer surrounding the egg. J Cell Biol. 1994;125(5):1157–1163. View this article via: PubMed CrossRef Google Scholar
- Baba D, et al. Mouse sperm lacking cell surface hyaluronidase PH-20 can pass through the layer of cumulus cells and fertilize the egg. J Biol Chem. 2002;277(33):30310–30314. View this article via: PubMed Google Scholar
- Kim E, Baba D, Kimura M, Yamashita M, Kashiwabara S, Baba T. Identification of a hyaluronidase, Hyal5, involved in penetration of mouse sperm through cumulus mass. Proc Natl Acad Sci U S A. 2005;102(50):18028–18033. View this article via: PubMed CrossRef Google Scholar
- Kimura M, et al. Functional roles of sperm hyaluronidases, HYAL5 and SPAM1, in fertilization in mice. Biol Reprod. 2009;81(5):939–94.
- Saling PM, Storey BT. Mouse gamete interactions during fertilization in vitro. Chlortetracycline as a fluorescent probe for the mouse sperm acrosome reaction. J Cell Biol. 1979;83(3):544–555. View this article via: PubMed CrossRef Google Scholar
- Cross NL, Meizel S. Methods for evaluating the acrosomal status of mammalian sperm. Biol Reprod. 1989;41(4):635–641. View this article via: PubMed CrossRef Google Scholar
- Lai YM, et al. Coculture of human spermatozoa with reproductive tract cell monolayers can enhance sperm functions better than coculture with Vero cell monolayers. J Assist Reprod Genet. 1996;13(5):417–422. View this article via: PubMed CrossRef Google Scholar
- Nakanishi T, et al. Real-time observation of acrosomal dispersal from mouse sperm using GFP as a marker protein. FEBS Lett. 1999;449(2–3):277–283. View this article via: PubMed CrossRef Google Scholar
- Hasuwa H, Muro Y, Ikawa M, Kato N, Tsujimoto Y, Okabe M. Transgenic mouse sperm that have green acrosome and red mitochondria allow visualization of sperm and their acrosome reaction in vivo. Exp Anim. 2010;59(1):105–107. View this article via: PubMed CrossRef Google Scholar
- Nakanishi T, Ikawa M, Yamada S, Toshimori K, Okabe M. Alkalinization of acrosome measured by GFP as a pH indicator and its relation to sperm capacitation. Dev Biol. 2001;237(1):222–231. View this article via: PubMed CrossRef Google Scholar
- Buffone MG, Foster JA, Gerton GL. The role of the acrosomal matrix in fertilization. Int J Dev Biol. 2008;52(5–6):511–522. View this article via: PubMed CrossRef Google Scholar
- Cherr GN, Lambert H, Meizel S, Katz DF. In vitro studies of the golden hamster sperm acrosome reaction: completion on the zona pellucida and induction by homologous soluble zonae pellucidae. Dev Biol. 1986;114(1):119–131. View this article via: PubMed CrossRef Google Scholar
- Florman HM, Storey BT. Mouse gamete interactions: the zona pellucida is the site of the acrosome reaction leading to fertilization in vitro. Dev Biol. 1982;91(1):121–130. View this article via: PubMed CrossRef Google Scholar
- Fukami K, et al. Requirement of phospholipase Cdelta4 for the zona pellucida-induced acrosome reaction. Science. 2001;292(5518):920–923. View this article via: PubMed CrossRef Google Scholar
- Baibakov B, Gauthier L, Talbot P, Rankin TL, Dean J. Sperm binding to the zona pellucida is not sufficient to induce acrosome exocytosis. Development. 2007;134(5):933–943. View this article via: PubMed CrossRef Google Scholar
- Yanagimachi R. Mammalian fertilization. In: Knobil E, Neill JD, eds. The Physiology of Reproduction. 2nd ed. New York, NY: Raven Press; 1994:189–317.
- Bedford JM, Mock OB, Goodman SM. Novelties of conception in insectivorous mammals (Lipotyphla), particularly shrews. Biol Rev Camb Philos Soc. 2004;79(4):891–909. View this article via: PubMed Google Scholar
- Patrat C, Serres C, Jouannet P. The acrosome reaction in human spermatozoa. Biol Cell. 2000;92(3–4):255–266. View this article via: PubMed Google Scholar
- Toyoda Y, Yokoyama M, Hoshi T. Studies on the fertilization of mouse eggs in vitro I: In vitro fertilization of eggs by fresh epididymal sperm [in Japanese]. Jpn J Anim Reprod. 1971;16:147–151.
- Batiz LF, et al. Sperm from hyh mice carrying a point mutation in alphaSNAP have a defect in acrosome reaction. PLoS One. 2009;4(3):e4963. View this article via: PubMed CrossRef Google Scholar
- Reim K, et al. Complexins regulate a late step in Ca2+-dependent neurotransmitter release. Cell. 2001;104(1):71–81. View this article via: PubMed Google Scholar
- Zhao L, Burkin HR, Shi X, Li L, Reim K, Miller DJ. Complexin I is required for mammalian sperm acrosomal exocytosis. Dev Biol. 2007;309(2):236–244. View this article via: PubMed CrossRef Google Scholar
- Bleil JD, Wassarman PM. Mammalian sperm-egg interaction: identification of a glycoprotein in mouse egg zonae pellucidae possessing receptor activity for sperm. Cell. 1980;20(3):873–882. View this article via: PubMed CrossRef Google Scholar
- Bleil JD, Wassarman PM. Sperm-egg interactions in the mouse: sequence of events and induction of the acrosome reaction by a zona pellucida glycoprotein. Dev Biol. 1983;95(2):317–324. View this article via: PubMed CrossRef Google Scholar
- Arnoult C, Zeng Y, Florman HM. ZP3-dependent activation of sperm cation channels regulates acrosomal secretion during mammalian fertilization. J Cell Biol. 1996;134(3):637–645. View this article via: PubMed CrossRef Google Scholar
- Bleil JD, Greve JM, Wassarman PM. Identification of a secondary sperm receptor in the mouse egg zona pellucida: role in maintenance of binding of acrosome-reacted sperm to eggs. Dev Biol. 1988;128(2):376–385. View this article via: PubMed Google Scholar
- Kurasawa S, Schultz RM, Kopf GS. Egg-induced modifications of the zona pellucida of mouse eggs: effects of microinjected inositol 1,4,5-trisphosphate. Dev Biol. 1989;133(1):295–304. View this article via: PubMed CrossRef Google Scholar
- Green DP. Three-dimensional structure of the zona pellucida. Rev Reprod. 1997;2(3):147–156. View this article via: PubMed Google Scholar
- Rankin T, Talbot P, Lee E, Dean J. Abnormal zonae pellucidae in mice lacking ZP1 result in early embryonic loss. Development. 1999;126(17):3847–3855. View this article via: PubMed Google Scholar
- Rankin TL, O’Brien M, Lee E, Wigglesworth K, Eppig J, Dean J. Defective zonae pellucidae in Zp2-null mice disrupt folliculogenesis, fertility and development. Development. 2001;128(7):1119–1126. View this article via: PubMed Google Scholar
- Liu C, et al. Targeted disruption of the mZP3 gene results in production of eggs lacking a zona pellucida and infertility in female mice. Proc Natl Acad Sci U S A. 1996;93(11):5431–5436. View this article via: PubMed CrossRef Google Scholar
- Rankin T, et al. Mice homozygous for an insertional mutation in the Zp3 gene lack a zona pellucida and are infertile. Development. 1996;122(9):2903–2910. View this article via: PubMed Google Scholar
- Lefievre L, et al. Four zona pellucida glycoproteins are expressed in the human. Hum Reprod. 2004;19(7):1580–1586. View this article via: PubMed Google Scholar
- Rankin TL, et al. Fertility and taxon-specific sperm binding persist after replacement of mouse sperm receptors with human homologs. Dev Cell. 2003;5(1):33–43. View this article via: PubMed Google Scholar
- Bleil JD, Wassarman PM. Galactose at the nonreducing terminus of O-linked oligosaccharides of mouse egg zona pellucida glycoprotein ZP3 is essential for the glycoprotein’s sperm receptor activity. Proc Natl Acad Sci U S A. 1988;85(18):6778–6782. View this article via: PubMed CrossRef Google Scholar
- Florman HM, Wassarman PM. O-linked oligosaccharides of mouse egg ZP3 account for its sperm receptor activity. Cell. 1985;41(1):313–324. View this article via: PubMed CrossRef Google Scholar
- Shur BD, Hall NG. A role for mouse sperm surface galactosyltransferase in sperm binding to the egg zona pellucida. J Cell Biol. 1982;95(2 Pt 1):574–579. View this article via: PubMed CrossRef Google Scholar
- Shi S, et al. Inactivation of the Mgat1 gene in oocytes impairs oogenesis, but embryos lacking complex and hybrid N-glycans develop and implant. Mol Cell Biol. 2004;24(22):9920–9929. View this article via: PubMed CrossRef Google Scholar
- Williams SA, Xia L, Cummings RD, McEver RP, Stanley P. Fertilization in mouse does not require terminal galactose or N-acetylglucosamine on the zona pellucida glycans. J Cell Sci. 2007;120(Pt 8):1341–1349. View this article via: PubMed CrossRef Google Scholar
- Lopez LC, Bayna EM, Litoff D, Shaper NL, Shaper JH, Shur BD. Receptor function of mouse sperm surface galactosyltransferase during fertilization. J Cell Biol. 1985;101(4):1501–1510. View this article via: PubMed CrossRef Google Scholar
- Hall L, Frayne J. Non-functional fertility genes in humans: contributory factors in reduced male fertility? Hum Fertil (Camb). 1999;2(1):36–41. View this article via: PubMed CrossRef Google Scholar
- Grzmil P, et al. Human cyritestin genes (CYRN1 and CYRN2) are non-functional. Biochem J. 2001;357(Pt 2):551–556. View this article via: PubMed Google Scholar
- Cheng A, et al. Sperm-egg recognition in the mouse: characterization of sp56, a sperm protein having specific affinity for ZP3. J Cell Biol. 1994;125(4):867–878. View this article via: PubMed CrossRef Google Scholar
- Bookbinder LH, Cheng A, Bleil JD. Tissue- and species-specific expression of sp56, a mouse sperm fertilization protein. Science. 1995;269(5220):86–89. View this article via: PubMed CrossRef Google Scholar
- Gao Z, Garbers DL. Species diversity in the structure of zonadhesin, a sperm-specific membrane protein containing multiple cell adhesion molecule-like domains. J Biol Chem. 1998;273(6):3415–3421. View this article via: PubMed CrossRef Google Scholar
- Ueda Y, et al. PGAP1 knock-out mice show otocephaly and male infertility. J Biol Chem. 2007;282(42):30373–30380. View this article via: PubMed Google Scholar
- Adham IM, Nayernia K, Engel W. Spermatozoa lacking acrosin protein show delayed fertilization. Mol Reprod Dev. 1997;46(3):370–376. View this article via: PubMed CrossRef Google Scholar
- Honda A, Yamagata K, Sugiura S, Watanabe K, Baba T. A mouse serine protease TESP5 is selectively included into lipid rafts of sperm membrane presumably as a glycosylphosphatidylinositol-anchored protein. J Biol Chem. 2002;277(19):16976–16984. View this article via: PubMed CrossRef Google Scholar
- Yamashita M, Honda A, Ogura A, Kashiwabara S, Fukami K, Baba T. Reduced fertility of mouse epididymal sperm lacking Prss21/Tesp5 is rescued by sperm exposure to uterine microenvironment. Genes Cells. 2008;13(10):1001–1013. View this article via: PubMed CrossRef Google Scholar
- Bedford JM. Ultrastructural changes in the sperm head during fertilization in the rabbit. Am J Anat. 1968;123(2):329–358. View this article via: PubMed CrossRef Google Scholar
- Stein KK, Primakoff P, Myles D. Sperm-egg fusion: events at the plasma membrane. J Cell Sci. 2004;117(Pt 26):6269–6274. View this article via: PubMed Google Scholar
- Okabe M, et al. Capacitation-related changes in antigen distribution on mouse sperm heads and its relation to fertilization rate in vitro. J Reprod Immunol. 1987;11(2):91–100. View this article via: PubMed CrossRef Google Scholar
- Sosnik J, et al. Tssk6 is required for Izumo relocalization and gamete fusion in the mouse. J Cell Sci. 2009;122(Pt 15):2741–2749. View this article via: PubMed Google Scholar
- Spiridonov NA, et al. Identification and characterization of SSTK, a serine/threonine protein kinase essential for male fertility. Mol Cell Biol. 2005;25(10):4250–4261. View this article via: PubMed Google Scholar
- Almeida EA, et al. Mouse egg integrin alpha 6 beta 1 functions as a sperm receptor. Cell. 1995;81(7):1095–1104. View this article via: PubMed Google Scholar
- Miller BJ, Georges-Labouesse E, Primakoff P, Myles DG. Normal fertilization occurs with eggs lacking the integrin alpha6beta1 and is CD9-dependent. J Cell Biol. 2000;149(6):1289–1296. View this article via: PubMed CrossRef Google Scholar
- Alfieri JA, Martin AD, Takeda J, Kondoh G, Myles DG, Primakoff P. Infertility in female mice with an oocyte-specific knockout of GPI-anchored proteins. J Cell Sci. 2003;116(Pt 11):2149–2155. View this article via: PubMed CrossRef Google Scholar
- Miyado K, et al. The fusing ability of sperm is bestowed by CD9-containing vesicles released from eggs in mice. Proc Natl Acad Sci U S A. 2008;105(35):12921–12926. View this article via: PubMed Google Scholar
- Yamagata K, et al. Acrosin accelerates the dispersal of sperm acrosomal proteins during acrosome reaction. J Biol Chem. 1998;273(17):10470–10474. View this article via: PubMed CrossRef Google Scholar
- Yan C, et al. Synergistic roles of bone morphogenetic protein 15 and growth differentiation factor 9 in ovarian function. Mol Endocrinol. 2001;15(6):854–866. View this article via: PubMed CrossRef Google Scholar
- Inoue N, et al. Disruption of mouse CD46 causes an accelerated spontaneous acrosome reaction in sperm. Mol Cell Biol. 2003;23(7):2614–2622. View this article via: PubMed CrossRef Google Scholar
- Rubinstein E, et al. Reduced fertility of female mice lacking CD81. Dev Biol. 2006;290(2):351–358. View this article via: PubMed CrossRef Google Scholar
- Da Ros VG, et al. Impaired sperm fertilizing ability in mice lacking Cysteine-RIch Secretory Protein 1 (CRISP1). Dev Biol. 2008;320(1):12–18. View this article via: PubMed CrossRef Google Scholar
- Kohn MJ, Sztein J, Yagi R, Depamphilis ML, Kaneko KJ. The acrosomal protein Dickkopf-like 1 (DKKL1) facilitates sperm penetration of the zona pellucida [published online ahead of print July 9, 2009]. Fertil Steril. doi:10.1016/j.fertnstert.2009.06.010. View this article via: PubMed Google Scholar
- Dong J, Albertini DF, Nishimori K, Kumar TR, Lu N, Matzuk MM. Growth differentiation factor-9 is required during early ovarian folliculogenesis. Nature. 1996;383(6600):531–535. View this article via: PubMed CrossRef Google Scholar
- Hellsten E, Evans JP, Bernard DJ, Janne PA, Nussbaum RL. Disrupted sperm function and fertilin beta processing in mice deficient in the inositol polyphosphate 5-phosphatase Inpp5b. Dev Biol. 2001;240(2):641–653. View this article via: PubMed Google Scholar
- Ensslin MA, Shur BD. Identification of mouse sperm SED1, a bimotif EGF repeat and discoidin-domain protein involved in sperm-egg binding. Cell. 2003;114(4):405–417. View this article via: PubMed CrossRef Google Scholar
- Gyamera-Acheampong C, et al. Sperm from mice genetically deficient for the PCSK4 proteinase exhibit accelerated capacitation, precocious acrosome reaction, reduced binding to egg zona pellucida, and impaired fertilizing ability. Biol Reprod. 2006;74(4):666–673. View this article via: PubMed Google Scholar
- Sutton KA, Jungnickel MK, Florman HM. A polycystin-1 controls postcopulatory reproductive selection in mice. Proc Natl Acad Sci U S A. 2008;105(25):8661–8666. View this article via: PubMed Google Scholar
- Hizaki H, et al. Abortive expansion of the cumulus and impaired fertility in mice lacking the prostaglandin E receptor subtype EP(2). Proc Natl Acad Sci U S A. 1999;96(18):10501–10506. View this article via: PubMed CrossRef Google Scholar
- Lim H, et al. Multiple female reproductive failures in cyclooxygenase 2-deficient mice. Cell. 1997;91(2):197–208. View this article via: PubMed Google Scholar
- Nayernia K, et al. Asthenozoospermia in mice with targeted deletion of the sperm mitochondrion-associated cysteine-rich protein (Smcp) gene. Mol Cell Biol. 2002;22(9):3046–3052. View this article via: PubMed CrossRef Google Scholar
- Zhou CX, et al. An epididymis-specific beta-defensin is important for the initiation of sperm maturation. Nat Cell Biol. 2004;6(5):458–464. View this article via: PubMed CrossRef Google Scholar
- Hardy DM, Garbers DL. A sperm membrane protein that binds in a species-specific manner to the egg extracellular matrix is homologous to von Willebrand factor. J Biol Chem. 1995;270(44):26025–26028. View this article via: PubMed CrossRef Google Scholar
- Lin YN, Roy A, Yan W, Burns KH, Matzuk MM. Loss of zona pellucida binding proteins in the acrosomal matrix disrupts acrosome biogenesis and sperm morphogenesis. Mol Cell Biol. 2007;27(19):6794–6805. View this article via: CrossRef PubMed Google Scholar
- Version 1 (April 1, 2010): No description
Article tools
- Download citation information
- Send a comment
- Terms of use
- Standard abbreviations
- Need help? Email the journal
Review Series
Reproductive biology.
Uterine disorders and pregnancy complications: insights from mouse models Hyunjung Jade Lim et al.
The placenta: transcriptional, epigenetic, and physiological integration during development Emin Maltepe et al.
Making the blastocyst: lessons from the mouse Katie Cockburn et al.
Portrait of an oocyte: our obscure origin Roger Gosden et al.
The key role of vitamin A in spermatogenesis Cathryn A. Hogarth et al.
The ovary: basic biology and clinical implications JoAnne S. Richards et al.
Fertilization: a sperm’s journey to and interaction with the oocyte Masahito Ikawa et al.
How we are born Sudhansu K. Dey
- Article usage
- Citations to this article
- Introduction
- Sperm migration in the female reproductive tract
- Sperm-cumulus interaction
- Acrosome reaction
- Sperm proteins required for ZP binding
- ZP penetration
- Sperm-egg fusion
- Conclusions
- Acknowledgments
- Version history

Sign up for email alerts
share this!
May 21, 2024
This article has been reviewed according to Science X's editorial process and policies . Editors have highlighted the following attributes while ensuring the content's credibility:
fact-checked
peer-reviewed publication
trusted source
Study finds widespread 'cell cannibalism' and related phenomena across tree of life
by Richard Harth, Arizona State University
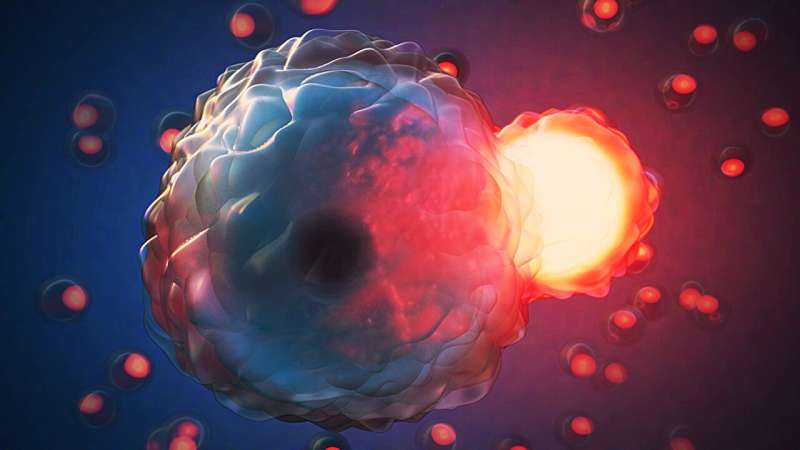
In a new review paper, Carlo Maley and Arizona State University colleagues describe cell-in-cell phenomena in which one cell engulfs and sometimes consumes another. The study shows that cases of this behavior, including cell cannibalism, are widespread across the tree of life.
The findings challenge the common perception that cell-in-cell events are largely restricted to cancer cells. Rather, these events appear to be common across diverse organisms, from single-celled amoebas to complex multicellular animals.
The widespread occurrence of such interactions in non-cancer cells suggests that these events are not inherently "selfish" or "cancerous" behaviors. Rather, the researchers propose that cell-in-cell phenomena may play crucial roles in normal development , homeostasis and stress response across a wide range of organisms.
The study argues that targeting cell-in-cell events as an approach to treating cancer should be abandoned, as these phenomena are not unique to malignancy.
By demonstrating that occurrences span a wide array of life forms and are deeply rooted in our genetic makeup , the research invites us to reconsider fundamental concepts of cellular cooperation, competition and the intricate nature of multicellularity. The study opens new avenues for research in evolutionary biology , oncology and regenerative medicine.
The research , published in Scientific Reports , is the first to systematically investigate cell-in-cell phenomena across the tree of life. The group's findings could help redefine the understanding of cellular behavior and its implications for multicellularity, cancer and the evolutionary journey of life itself.
"We first got into this work because we learned that cells don't just compete for resources—they actively kill and eat each other," Maley says. "That's a fascinating aspect of the ecology of cancer cells . But further exploration revealed that these phenomena happen in normal cells, and sometimes neither cell dies, resulting in an entirely new type of hybrid cell."
Maley is a researcher with the Biodesign Center for Biocomputing, Security and Society; professor in the School of Life Sciences at ASU; and director of the Arizona Cancer Evolution Center.
The study was conducted in collaboration with first author Stefania E. Kapsetaki, formerly with ASU and now a researcher at Tufts University, and Luis Cisneros, formerly with ASU and currently a researcher at Mayo Clinic.
From selfish to cooperative cell interactions
Cell-in-cell events have long been observed but remain poorly understood, especially outside the context of immune responses or cancer. The earliest genes responsible for cell-in-cell behavior date back over 2 billion years, suggesting the phenomena play an important—though yet-to-be-determined—role in living organisms. Understanding the diverse functions of cell-in-cell events, both in normal physiology and disease, is important for developing more effective cancer therapies.
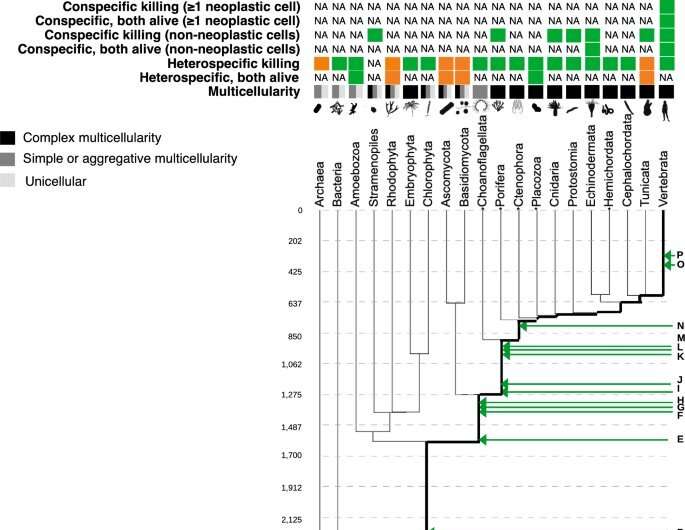
The review delves into the occurrence, genetic underpinnings and evolutionary history of cell-in-cell phenomena, shedding light on a behavior once thought to be an anomaly. The researchers reviewed more than 500 articles to catalog the various forms of cell-in-cell phenomena observed across the tree of life.
The study describes 16 different taxonomic groups in which cell-in-cell behavior is found to occur. The cell-in-cell events were classified into six distinct categories based on the degree of relatedness between the host and prey cells, as well as the outcome of the interaction (whether one or both cells survived).
A spectrum of cell-in-cell behaviors are highlighted in the study, ranging from completely selfish acts, where one cell kills and consumes another, to more cooperative interactions, where both cells remain alive. For example, the researchers found evidence of "heterospecific killing," where a cell engulfs and kills a cell of a different species, across a wide range of unicellular, facultatively multicellular, and obligate multicellular organisms. In contrast, "conspecific killing," where a cell consumes another cell of the same species, was less common, observed in only three of the seven major taxonomic groups examined.
Obligate multicellular organisms are those that must exist in a multicellular form throughout their life cycle. They cannot survive or function as single cells. Examples include most animals and plants. Facultative multicellular organisms are organisms that can exist either as single cells or in a multicellular form depending on environmental conditions. For example, certain types of algae may live as single cells in some conditions but form multicellular colonies in others.
The team also documented cases of cell-in-cell phenomena where both the host and prey cells remained alive after the interaction, suggesting these events may serve important biological functions beyond just killing competitors.
"Our categorization of cell-in-cell phenomena across the tree of life is important for better understanding the evolution and mechanism of these phenomena," Kapsetaki says. "Why and how exactly do they happen? This is a question that requires further investigation across millions of living organisms, including organisms where cell-in-cell phenomena may not yet have been searched for."
Ancient genes
In addition to cataloging the diverse cell-in-cell behaviors, the researchers also investigated the evolutionary origins of the genes involved in these processes. Surprisingly, they found that many of the key cell-in-cell genes emerged long before the evolution of obligate multicellularity.
"When we look at genes associated with known cell-in-cell mechanisms in species that diverged from the human lineage a very long time ago, it turns out that the human orthologs (genes that evolved from a common ancestral gene) are typically associated with normal functions of multicellularity, like immune surveillance," Cisneros says.
In total, 38 genes associated with cell-in-cell phenomena were identified, and 14 of these originated over 2.2 billion years ago, predating the common ancestor of some facultatively multicellular organisms. This suggests that the molecular machinery for cell cannibalism evolved before the major transitions to complex multicellularity.
The ancient cell-in-cell genes identified in the study are involved in a variety of cellular processes, including cell–cell adhesion, phagocytosis (engulfment), intracellular killing of pathogens and regulation of energy metabolism. This diversity of functions indicates that cell-in-cell events likely served important roles even in single-celled and simple multicellular organisms well before the emergence of complex multicellular life.
Journal information: Scientific Reports
Provided by Arizona State University
Explore further
Feedback to editors

Florida fossil porcupine solves a prickly dilemma 10 million years in the making
13 minutes ago

GPT's inaccuracies in agriculture could lead to crop losses and food crises
17 minutes ago

Health risks from global warming can help drive city climate action, study finds
19 minutes ago

Bacterial model helps reveal how our bodies prevent population explosions—and cancer
32 minutes ago

Tropical forest resilience to seasonal drought linked to nutrient availability
44 minutes ago

Salty soil sensitizes plants to an unconventional mode of bacterial toxicity

Study introduces a cleaner way to produce ammonia at room temperature and pressure

A change of direction: Research reveals a new method to manipulate cell movement in embryos

New approach enhances accelerator's capability to uncover clues from supernovae in lunar dust

New molecule found to suppress bacterial antibiotic resistance evolution
Relevant physicsforums posts, looking for today's dna knowledge.
17 hours ago
A DNA Animation
18 hours ago
Covid Vaccines Reducing Infections
May 27, 2024
Human Sperm, Egg Cells Mass-Generated using iPS
And now, here comes covid-19 version ba.2, ba.4, ba.5,....
May 25, 2024
DNA-maternity test - could you see other relationship than mother?
May 19, 2024
More from Biology and Medical
Related Stories

Macroalgae genetics study sheds light on how seaweed became multicellular
Apr 12, 2024

Why eukaryotes, not bacteria, evolved complex multicellularity
Mar 13, 2024

Team pieces together the mystery of how single cell life forms evolved into multicellular organisms
Feb 2, 2018

Algae in Swedish lakes provide insights to how complex life on Earth developed
Apr 24, 2023

Evolution and ecological competition of multicellular life cycles
Sep 14, 2022

Billion-year-old fossil reveals missing link in the evolution of animals
Apr 29, 2021
Recommended for you

New research shows soil microorganisms could produce additional greenhouse gas emissions from thawing permafrost

How killifish embryos use suspended animation to survive over 8 months of drought

Discovery of ancient rock impression suggests ability to form cornified skin goes back to early evolution of tetrapods
2 hours ago

Viper-mimicking snake from Asia is a unique branch in the reptile evolutionary tree
Let us know if there is a problem with our content.
Use this form if you have come across a typo, inaccuracy or would like to send an edit request for the content on this page. For general inquiries, please use our contact form . For general feedback, use the public comments section below (please adhere to guidelines ).
Please select the most appropriate category to facilitate processing of your request
Thank you for taking time to provide your feedback to the editors.
Your feedback is important to us. However, we do not guarantee individual replies due to the high volume of messages.
E-mail the story
Your email address is used only to let the recipient know who sent the email. Neither your address nor the recipient's address will be used for any other purpose. The information you enter will appear in your e-mail message and is not retained by Phys.org in any form.
Newsletter sign up
Get weekly and/or daily updates delivered to your inbox. You can unsubscribe at any time and we'll never share your details to third parties.
More information Privacy policy
Donate and enjoy an ad-free experience
We keep our content available to everyone. Consider supporting Science X's mission by getting a premium account.
E-mail newsletter

IMAGES
VIDEO
COMMENTS
These sperm cells then travel through a series of ducts within the testes, called the rete testis and the epididymis, where they undergo further maturation and acquire the ability to swim. ... The journey of sperm through the reproductive system. Sperm Production: The journey begins in the testes, where sperm are produced through a process ...
The journey through the female reproductive system. In the process of ejaculation, sperm cells leave the man and enter the vagina. This is where the sperm cells begin the second part of their journey to fertilization. During this second part of the journey the sperm again encounter an large number of obstacles.
Sperm are haploid; they contain one set of 23 chromosomes. They are created by the cellular division process known as meiosis, which creates 4 sperm from a single germ cell. They're also very small, only about 50μm long. Sperm are ejaculated in semen, a basic fluid with a pH of about 7.4. The sperm's target is the egg.
A sperm cell or spermatozoon is a gamete (sex cell) produced in the male reproductive tract. It is a motile cell with a single aim - to fertilize a female egg. Each sperm cell contains the entire genome of the male that produces it. In combination with the female genome contained within the egg, a zygote is formed - a single totipotent stem ...
The pathway of sperm is the journey that sperm cells take from the testes to the female reproductive tract during fertilization. The journey involves a series of steps, including production, maturation, and transport. Sperm cells are produced in the testes through a process called spermatogenesis that begins at puberty and continues throughout ...
Spermatogenesis. Discover the vital role of the testes in the male reproductive system. Learn about the production of sperm and testosterone, the journey of sperm through the seminiferous tubules, and the process of spermatogenesis. Explore the importance of the sertoli and leydig cells, and the unique function of the epididymis.
spermatogenesis, the origin and development of the sperm cells within the male reproductive organs, the testes.The testes are composed of numerous thin tightly coiled tubules known as the seminiferous tubules; the sperm cells are produced within the walls of the tubules. Within the walls of the tubules, also, are many randomly scattered cells, called Sertoli cells, that function to support and ...
Fertilization: Sperm Penetrates Egg. 5 /9. It takes about 24 hours for a sperm cell to fertilize an egg. When the sperm penetrates the egg, the surface of the egg changes so that no other sperm ...
Mammalian sperm have to travel a long distance through the female reproductive tract to the oviduct, in which fertilization takes place. To accomplish the journey to the egg, sperm are equipped to overcome various obstacles that lie ahead, such as navigating the uterotubal junction (UTJ) and penetrating the egg extracellular matrices.
Egg, sperm, and fertilization. Sperm and egg cells are special sex cells for reproduction. Sperm cells are small, have a tail for movement, and carry male genetic material. The egg is large, round, and has a protective layer called the zona pellucida. During fertilization, sperm meets egg, and their genetic material combines.
Sperm are unique cells, produced through the complex and precisely orchestrated process of spermatogenesis, in which there are a number of checkpoints in place to guarantee delivery of a high ...
The Seven-Up sperm pathway provides insight into the intricate journey that sperm cells undertake during their development and maturation. Understanding the steps involved in spermatogenesis, particularly this specialized pathway, can shed light on various reproductive issues and potentially lead to novel interventions for infertility. So, the ...
This 3D animation shows the magic that happens when a sperm meets an egg and the sparks fly. Fertilization happens when a sperm cell successfully meets an eg...
Video summary. This short film uses CGI footage to help give a visual description of the process of human fertilisation, following the journey of both sperm and egg.
Let' uncover the fascinating science behind male reproduction, exploring the basics of anatomy of the male reproductive system, puberty, the ejaculation mech...
@doctorpedia. take a mesmerizing voyage into the journey of sperm cells, uncovering the astounding narrative of their creation and their incredible mission. ...
The remarkable journey that successful sperm take to reach an oocyte is long and tortuous, and includes movement through viscous fluid, avoiding dead ends and hostile immune cells. The privileged collection of sperm that complete this journey must pass selection steps in the vagina, cervix, uterus, utero-tubal junction, and oviduct.
This is a cordlike structure where the sperm mature and are stored. The release process starts when the penis fills with blood and becomes erect. Continuing to stimulate the penis will cause an ejaculation. Mature sperm begin their journey by travelling from the epididymis to the vas deferens, which propels sperm forward with smooth muscle ...
Fertilization occurs when a sperm cell penetrates an egg and combines with the 23 chromosomes the egg contains. That forms a single cell of 46 chromosomes . If all goes well, a baby will be born 9 ...
A sperm cell (right) swims towards a human egg (artificially coloured). Credit: AJ Photo/Science Photo Library. The day when human sperm and eggs can be grown in the laboratory has inched a step ...
The remarkable journey that successful sperm take to reach an oocyte is long and tortuous, and includes movement through viscous fluid, avoiding dead ends and hostile immune cells. The privileged collection of sperm that complete this journey must pass selection steps in the vagina, cervix, uterus, utero-tubal junction and oviduct.
The fluids provide the sperm cells with nutrients. The mixture of sperm and fluids is called semen. ... Describe the journey of a sperm from testis to ovum. Tap 'Show answer' to see five points ...
Mammalian sperm have to travel a long distance through the female reproductive tract to the oviduct, in which fertilization takes place. To accomplish the journey to the egg, sperm are equipped to overcome various obstacles that lie ahead, such as navigating the uterotubal junction (UTJ) and penetrating the egg extracellular matrices.
The remarkable journey that successful sperm take to reach an oocyte is long and tortuous, and includes movement through viscous fluid, avoiding dead ends and hostile immune cells. The privileged collection of sperm that complete this journey must pass selection steps in the vagina, cervix, uterus, utero-tubal junction and oviduct.
The study shows that cases of this behavior, including cell cannibalism, are widespread across the tree of life. The findings challenge the common perception that cell-in-cell events are largely ...