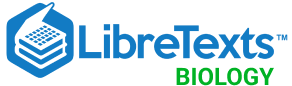
- school Campus Bookshelves
- menu_book Bookshelves
- perm_media Learning Objects
- login Login
- how_to_reg Request Instructor Account
- hub Instructor Commons

Margin Size
- Download Page (PDF)
- Download Full Book (PDF)
- Periodic Table
- Physics Constants
- Scientific Calculator
- Reference & Cite
- Tools expand_more
- Readability
selected template will load here
This action is not available.
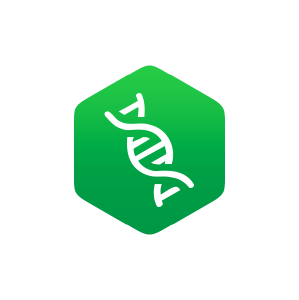
42.2: The Mechanism of Nerve Impulse Transmission
- Last updated
- Save as PDF
- Page ID 74335
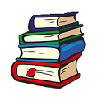
\( \newcommand{\vecs}[1]{\overset { \scriptstyle \rightharpoonup} {\mathbf{#1}} } \)
\( \newcommand{\vecd}[1]{\overset{-\!-\!\rightharpoonup}{\vphantom{a}\smash {#1}}} \)
\( \newcommand{\id}{\mathrm{id}}\) \( \newcommand{\Span}{\mathrm{span}}\)
( \newcommand{\kernel}{\mathrm{null}\,}\) \( \newcommand{\range}{\mathrm{range}\,}\)
\( \newcommand{\RealPart}{\mathrm{Re}}\) \( \newcommand{\ImaginaryPart}{\mathrm{Im}}\)
\( \newcommand{\Argument}{\mathrm{Arg}}\) \( \newcommand{\norm}[1]{\| #1 \|}\)
\( \newcommand{\inner}[2]{\langle #1, #2 \rangle}\)
\( \newcommand{\Span}{\mathrm{span}}\)
\( \newcommand{\id}{\mathrm{id}}\)
\( \newcommand{\kernel}{\mathrm{null}\,}\)
\( \newcommand{\range}{\mathrm{range}\,}\)
\( \newcommand{\RealPart}{\mathrm{Re}}\)
\( \newcommand{\ImaginaryPart}{\mathrm{Im}}\)
\( \newcommand{\Argument}{\mathrm{Arg}}\)
\( \newcommand{\norm}[1]{\| #1 \|}\)
\( \newcommand{\Span}{\mathrm{span}}\) \( \newcommand{\AA}{\unicode[.8,0]{x212B}}\)
\( \newcommand{\vectorA}[1]{\vec{#1}} % arrow\)
\( \newcommand{\vectorAt}[1]{\vec{\text{#1}}} % arrow\)
\( \newcommand{\vectorB}[1]{\overset { \scriptstyle \rightharpoonup} {\mathbf{#1}} } \)
\( \newcommand{\vectorC}[1]{\textbf{#1}} \)
\( \newcommand{\vectorD}[1]{\overrightarrow{#1}} \)
\( \newcommand{\vectorDt}[1]{\overrightarrow{\text{#1}}} \)
\( \newcommand{\vectE}[1]{\overset{-\!-\!\rightharpoonup}{\vphantom{a}\smash{\mathbf {#1}}}} \)
Skills to Develop
- Describe the basis of the resting membrane potential
- Explain the stages of an action potential and how action potentials are propagated
- Explain the similarities and differences between chemical and electrical synapses
- Describe long-term potentiation and long-term depression
All functions performed by the nervous system—from a simple motor reflex to more advanced functions like making a memory or a decision—require neurons to communicate with one another. While humans use words and body language to communicate, neurons use electrical and chemical signals. Just like a person in a committee, one neuron usually receives and synthesizes messages from multiple other neurons before “making the decision” to send the message on to other neurons.
Nerve Impulse Transmission within a Neuron
For the nervous system to function, neurons must be able to send and receive signals. These signals are possible because each neuron has a charged cellular membrane (a voltage difference between the inside and the outside), and the charge of this membrane can change in response to neurotransmitter molecules released from other neurons and environmental stimuli. To understand how neurons communicate, one must first understand the basis of the baseline or ‘resting’ membrane charge.
Neuronal Charged Membranes
The lipid bilayer membrane that surrounds a neuron is impermeable to charged molecules or ions. To enter or exit the neuron, ions must pass through special proteins called ion channels that span the membrane. Ion channels have different configurations: open, closed, and inactive, as illustrated in Figure \(\PageIndex{1}\). Some ion channels need to be activated in order to open and allow ions to pass into or out of the cell. These ion channels are sensitive to the environment and can change their shape accordingly. Ion channels that change their structure in response to voltage changes are called voltage-gated ion channels. Voltage-gated ion channels regulate the relative concentrations of different ions inside and outside the cell. The difference in total charge between the inside and outside of the cell is called the membrane potential .
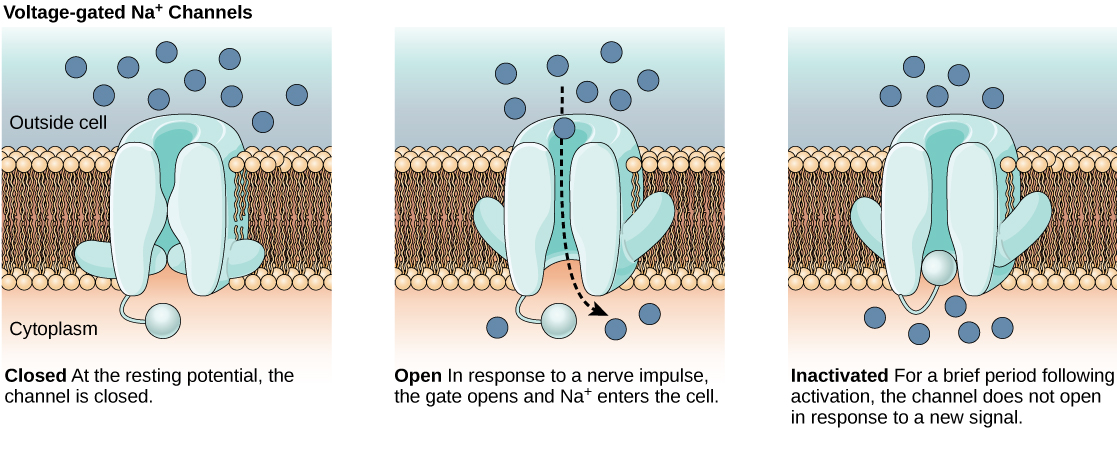
Link to Learning
This video discusses the basis of the resting membrane potential.
Resting Membrane Potential
A neuron at rest is negatively charged: the inside of a cell is approximately 70 millivolts more negative than the outside (−70 mV, note that this number varies by neuron type and by species). This voltage is called the resting membrane potential; it is caused by differences in the concentrations of ions inside and outside the cell. If the membrane were equally permeable to all ions, each type of ion would flow across the membrane and the system would reach equilibrium. Because ions cannot simply cross the membrane at will, there are different concentrations of several ions inside and outside the cell, as shown in the table below. The difference in the number of positively charged potassium ions (K + ) inside and outside the cell dominates the resting membrane potential (Figure \(\PageIndex{2}\)). When the membrane is at rest, K + ions accumulate inside the cell due to a net movement with the concentration gradient. The negative resting membrane potential is created and maintained by increasing the concentration of cations outside the cell (in the extracellular fluid) relative to inside the cell (in the cytoplasm). The negative charge within the cell is created by the cell membrane being more permeable to potassium ion movement than sodium ion movement. In neurons, potassium ions are maintained at high concentrations within the cell while sodium ions are maintained at high concentrations outside of the cell. The cell possesses potassium and sodium leakage channels that allow the two cations to diffuse down their concentration gradient. However, the neurons have far more potassium leakage channels than sodium leakage channels. Therefore, potassium diffuses out of the cell at a much faster rate than sodium leaks in. Because more cations are leaving the cell than are entering, this causes the interior of the cell to be negatively charged relative to the outside of the cell. The actions of the sodium potassium pump help to maintain the resting potential, once established. Recall that sodium potassium pumps brings two K + ions into the cell while removing three Na + ions per ATP consumed. As more cations are expelled from the cell than taken in, the inside of the cell remains negatively charged relative to the extracellular fluid. It should be noted that calcium ions (Cl – ) tend to accumulate outside of the cell because they are repelled by negatively-charged proteins within the cytoplasm.
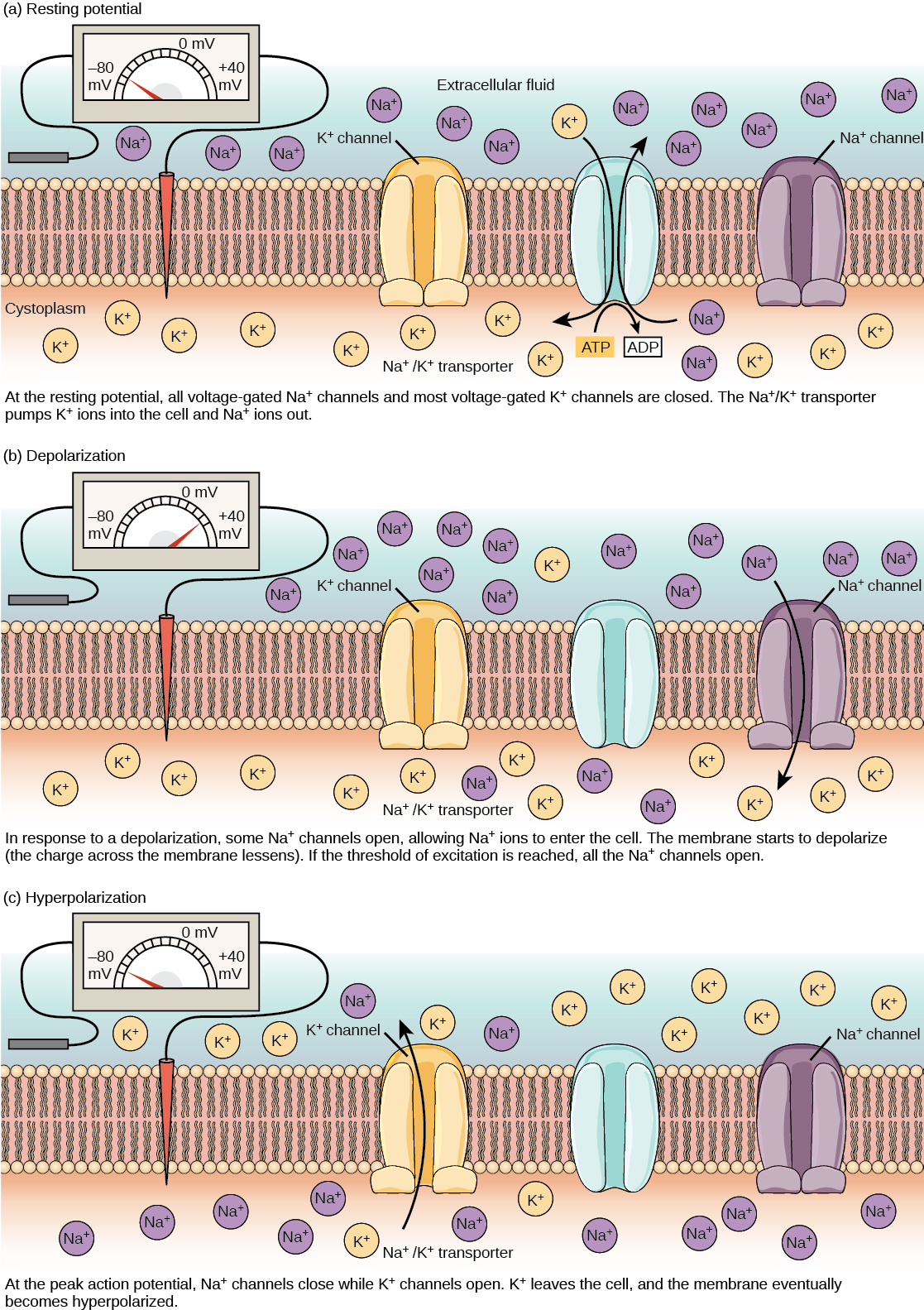
Action Potential
A neuron can receive input from other neurons and, if this input is strong enough, send the signal to downstream neurons. Transmission of a signal between neurons is generally carried by a chemical called a neurotransmitter. Transmission of a signal within a neuron (from dendrite to axon terminal) is carried by a brief reversal of the resting membrane potential called an action potential . When neurotransmitter molecules bind to receptors located on a neuron’s dendrites, ion channels open. At excitatory synapses, this opening allows positive ions to enter the neuron and results in depolarization of the membrane—a decrease in the difference in voltage between the inside and outside of the neuron. A stimulus from a sensory cell or another neuron depolarizes the target neuron to its threshold potential (-55 mV). Na + channels in the axon hillock open, allowing positive ions to enter the cell (Figure \(\PageIndex{3}\) and Figure \(\PageIndex{4}\)). Once the sodium channels open, the neuron completely depolarizes to a membrane potential of about +40 mV. Action potentials are considered an "all-or nothing" event, in that, once the threshold potential is reached, the neuron always completely depolarizes. Once depolarization is complete, the cell must now "reset" its membrane voltage back to the resting potential. To accomplish this, the Na + channels close and cannot be opened. This begins the neuron's refractory period , in which it cannot produce another action potential because its sodium channels will not open. At the same time, voltage-gated K + channels open, allowing K + to leave the cell. As K + ions leave the cell, the membrane potential once again becomes negative. The diffusion of K + out of the cell actually hyperpolarizes the cell, in that the membrane potential becomes more negative than the cell's normal resting potential. At this point, the sodium channels will return to their resting state, meaning they are ready to open again if the membrane potential again exceeds the threshold potential. Eventually the extra K + ions diffuse out of the cell through the potassium leakage channels, bringing the cell from its hyperpolarized state, back to its resting membrane potential.
Art Connection
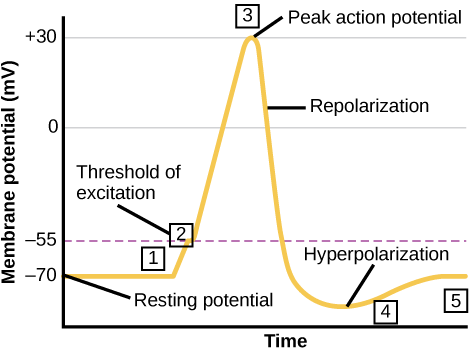
Potassium channel blockers, such as amiodarone and procainamide, which are used to treat abnormal electrical activity in the heart, called cardiac dysrhythmia, impede the movement of K + through voltage-gated K + channels. Which part of the action potential would you expect potassium channels to affect?
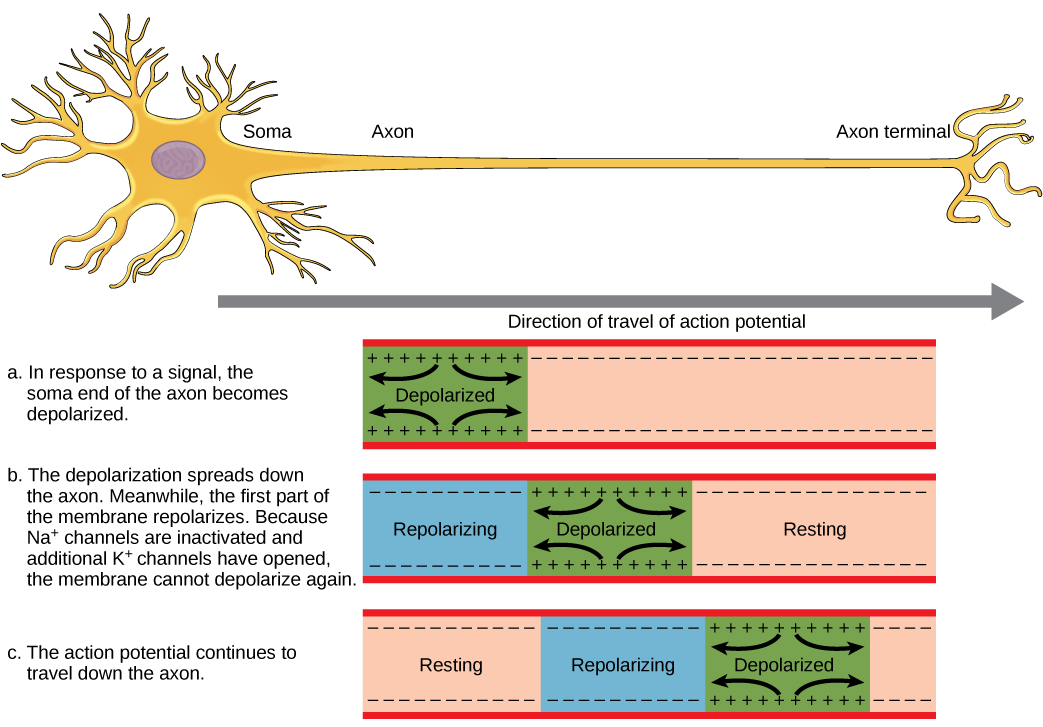
This video presents an overview of action potential.
Myelin and the Propagation of the Action Potential
For an action potential to communicate information to another neuron, it must travel along the axon and reach the axon terminals where it can initiate neurotransmitter release. The speed of conduction of an action potential along an axon is influenced by both the diameter of the axon and the axon’s resistance to current leak. Myelin acts as an insulator that prevents current from leaving the axon; this increases the speed of action potential conduction. In demyelinating diseases like multiple sclerosis, action potential conduction slows because current leaks from previously insulated axon areas. The nodes of Ranvier, illustrated in Figure \(\PageIndex{5}\) are gaps in the myelin sheath along the axon. These unmyelinated spaces are about one micrometer long and contain voltage gated Na + and K + channels. Flow of ions through these channels, particularly the Na + channels, regenerates the action potential over and over again along the axon. This ‘jumping’ of the action potential from one node to the next is called saltatory conduction . If nodes of Ranvier were not present along an axon, the action potential would propagate very slowly since Na + and K + channels would have to continuously regenerate action potentials at every point along the axon instead of at specific points. Nodes of Ranvier also save energy for the neuron since the channels only need to be present at the nodes and not along the entire axon.
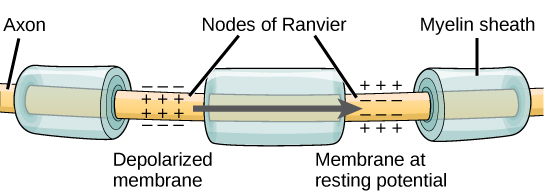
Synaptic Transmission
The synapse or “gap” is the place where information is transmitted from one neuron to another. Synapses usually form between axon terminals and dendritic spines, but this is not universally true. There are also axon-to-axon, dendrite-to-dendrite, and axon-to-cell body synapses. The neuron transmitting the signal is called the presynaptic neuron, and the neuron receiving the signal is called the postsynaptic neuron. Note that these designations are relative to a particular synapse—most neurons are both presynaptic and postsynaptic. There are two types of synapses: chemical and electrical.
Chemical Synapse
When an action potential reaches the axon terminal it depolarizes the membrane and opens voltage-gated Na + channels. Na + ions enter the cell, further depolarizing the presynaptic membrane. This depolarization causes voltage-gated Ca 2+ channels to open. Calcium ions entering the cell initiate a signaling cascade that causes small membrane-bound vesicles, called synaptic vesicles , containing neurotransmitter molecules to fuse with the presynaptic membrane. Synaptic vesicles are shown in Figure \(\PageIndex{6}\), which is an image from a scanning electron microscope.
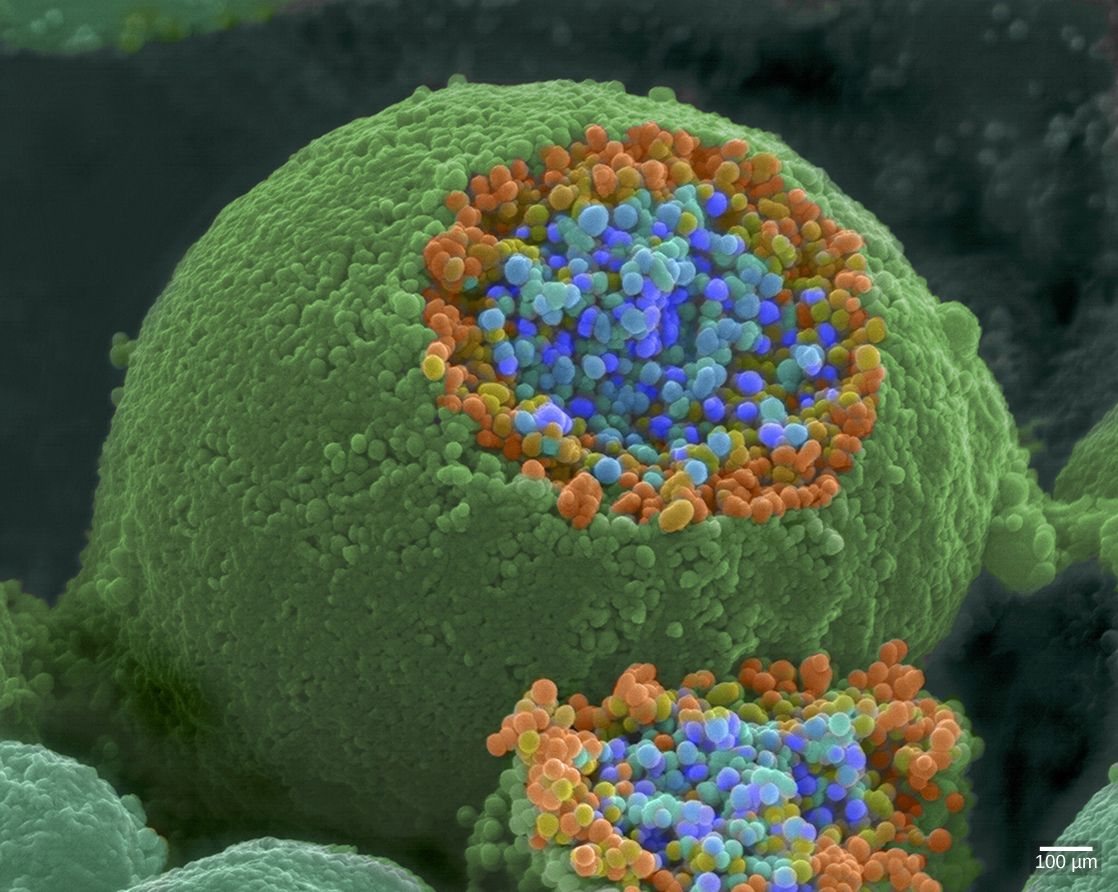
Fusion of a vesicle with the presynaptic membrane causes neurotransmitter to be released into the synaptic cleft , the extracellular space between the presynaptic and postsynaptic membranes, as illustrated in Figure \(\PageIndex{7}\). The neurotransmitter diffuses across the synaptic cleft and binds to receptor proteins on the postsynaptic membrane.
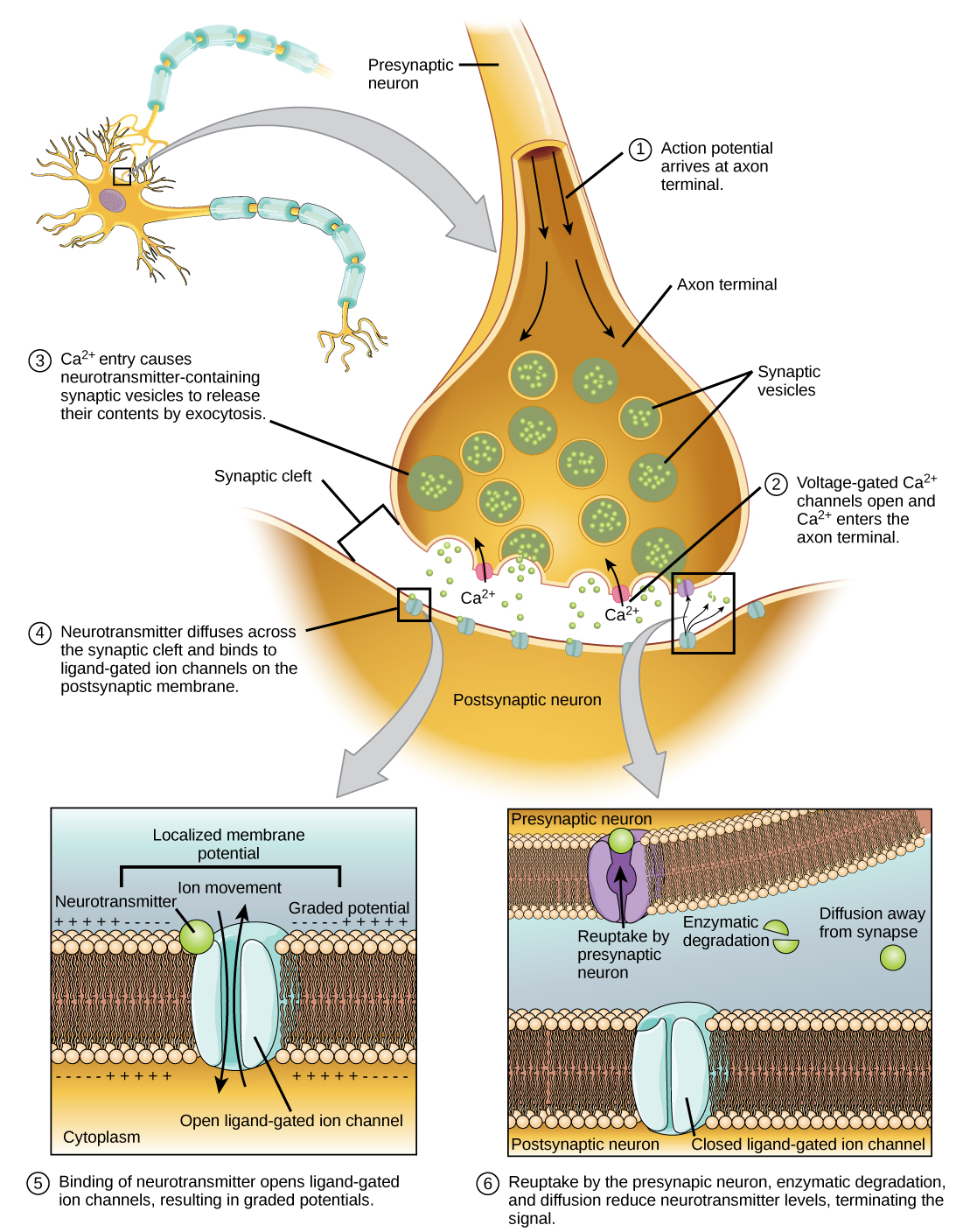
The binding of a specific neurotransmitter causes particular ion channels, in this case ligand-gated channels, on the postsynaptic membrane to open. Neurotransmitters can either have excitatory or inhibitory effects on the postsynaptic membrane, as detailed in the table below. For example, when acetylcholine is released at the synapse between a nerve and muscle (called the neuromuscular junction) by a presynaptic neuron, it causes postsynaptic Na + channels to open. Na + enters the postsynaptic cell and causes the postsynaptic membrane to depolarize. This depolarization is called an excitatory postsynaptic potential (EPSP) and makes the postsynaptic neuron more likely to fire an action potential. Release of neurotransmitter at inhibitory synapses causes inhibitory postsynaptic potentials (IPSPs) , a hyperpolarization of the presynaptic membrane. For example, when the neurotransmitter GABA (gamma-aminobutyric acid) is released from a presynaptic neuron, it binds to and opens Cl - channels. Cl - ions enter the cell and hyperpolarizes the membrane, making the neuron less likely to fire an action potential.
Once neurotransmission has occurred, the neurotransmitter must be removed from the synaptic cleft so the postsynaptic membrane can “reset” and be ready to receive another signal. This can be accomplished in three ways: the neurotransmitter can diffuse away from the synaptic cleft, it can be degraded by enzymes in the synaptic cleft, or it can be recycled (sometimes called reuptake) by the presynaptic neuron. Several drugs act at this step of neurotransmission. For example, some drugs that are given to Alzheimer’s patients work by inhibiting acetylcholinesterase, the enzyme that degrades acetylcholine. This inhibition of the enzyme essentially increases neurotransmission at synapses that release acetylcholine. Once released, the acetylcholine stays in the cleft and can continually bind and unbind to postsynaptic receptors.
Electrical Synapse
While electrical synapses are fewer in number than chemical synapses, they are found in all nervous systems and play important and unique roles. The mode of neurotransmission in electrical synapses is quite different from that in chemical synapses. In an electrical synapse, the presynaptic and postsynaptic membranes are very close together and are actually physically connected by channel proteins forming gap junctions. Gap junctions allow current to pass directly from one cell to the next. In addition to the ions that carry this current, other molecules, such as ATP, can diffuse through the large gap junction pores.
There are key differences between chemical and electrical synapses. Because chemical synapses depend on the release of neurotransmitter molecules from synaptic vesicles to pass on their signal, there is an approximately one millisecond delay between when the axon potential reaches the presynaptic terminal and when the neurotransmitter leads to opening of postsynaptic ion channels. Additionally, this signaling is unidirectional. Signaling in electrical synapses, in contrast, is virtually instantaneous (which is important for synapses involved in key reflexes), and some electrical synapses are bidirectional. Electrical synapses are also more reliable as they are less likely to be blocked, and they are important for synchronizing the electrical activity of a group of neurons. For example, electrical synapses in the thalamus are thought to regulate slow-wave sleep, and disruption of these synapses can cause seizures.
Signal Summation
Sometimes a single EPSP is strong enough to induce an action potential in the postsynaptic neuron, but often multiple presynaptic inputs must create EPSPs around the same time for the postsynaptic neuron to be sufficiently depolarized to fire an action potential. This process is called summation and occurs at the axon hillock, as illustrated in Figure \(\PageIndex{8}\). Additionally, one neuron often has inputs from many presynaptic neurons—some excitatory and some inhibitory—so IPSPs can cancel out EPSPs and vice versa. It is the net change in postsynaptic membrane voltage that determines whether the postsynaptic cell has reached its threshold of excitation needed to fire an action potential. Together, synaptic summation and the threshold for excitation act as a filter so that random “noise” in the system is not transmitted as important information.
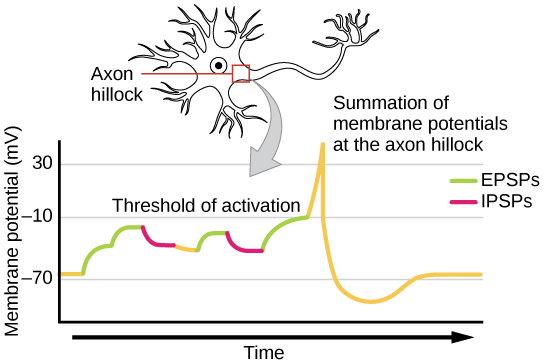
Everyday Connection: Brain-computer interface
Amyotrophic lateral sclerosis (ALS, also called Lou Gehrig’s Disease) is a neurological disease characterized by the degeneration of the motor neurons that control voluntary movements. The disease begins with muscle weakening and lack of coordination and eventually destroys the neurons that control speech, breathing, and swallowing; in the end, the disease can lead to paralysis. At that point, patients require assistance from machines to be able to breathe and to communicate. Several special technologies have been developed to allow “locked-in” patients to communicate with the rest of the world. One technology, for example, allows patients to type out sentences by twitching their cheek. These sentences can then be read aloud by a computer.
A relatively new line of research for helping paralyzed patients, including those with ALS, to communicate and retain a degree of self-sufficiency is called brain-computer interface (BCI) technology and is illustrated in Figure \(\PageIndex{9}\). This technology sounds like something out of science fiction: it allows paralyzed patients to control a computer using only their thoughts. There are several forms of BCI. Some forms use EEG recordings from electrodes taped onto the skull. These recordings contain information from large populations of neurons that can be decoded by a computer. Other forms of BCI require the implantation of an array of electrodes smaller than a postage stamp in the arm and hand area of the motor cortex. This form of BCI, while more invasive, is very powerful as each electrode can record actual action potentials from one or more neurons. These signals are then sent to a computer, which has been trained to decode the signal and feed it to a tool—such as a cursor on a computer screen. This means that a patient with ALS can use e-mail, read the Internet, and communicate with others by thinking of moving his or her hand or arm (even though the paralyzed patient cannot make that bodily movement). Recent advances have allowed a paralyzed locked-in patient who suffered a stroke 15 years ago to control a robotic arm and even to feed herself coffee using BCI technology.
Despite the amazing advancements in BCI technology, it also has limitations. The technology can require many hours of training and long periods of intense concentration for the patient; it can also require brain surgery to implant the devices.
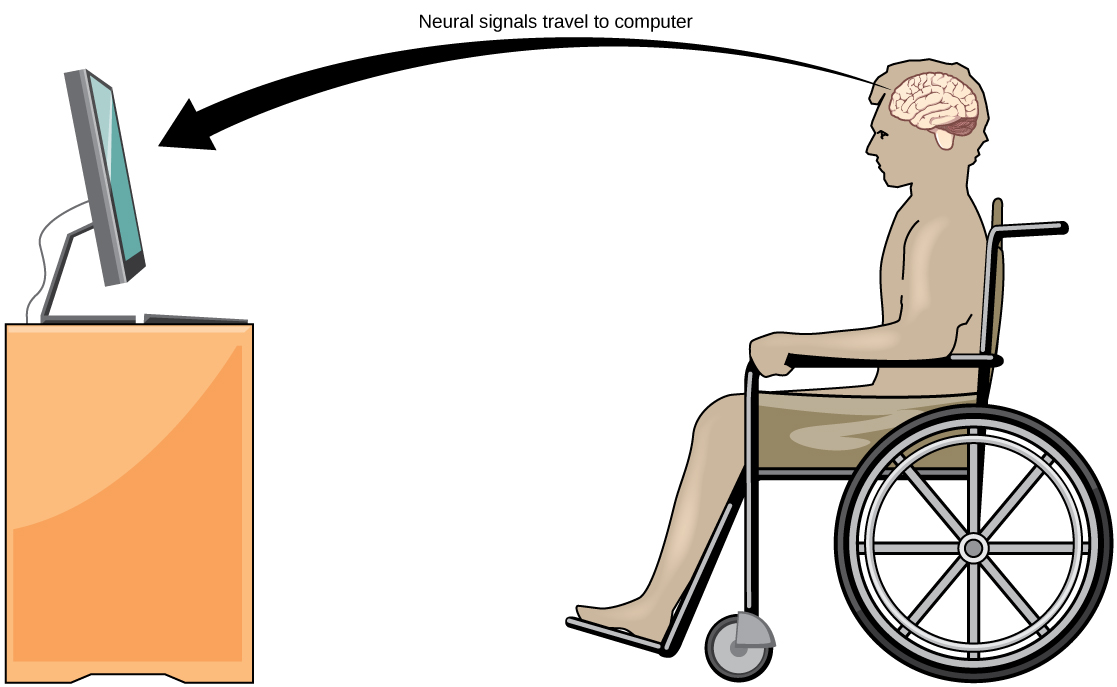
Watch this video in which a paralyzed woman use a brain-controlled robotic arm to bring a drink to her mouth, among other images of brain-computer interface technology in action.
Synaptic Plasticity
Synapses are not static structures. They can be weakened or strengthened. They can be broken, and new synapses can be made. Synaptic plasticity allows for these changes, which are all needed for a functioning nervous system. In fact, synaptic plasticity is the basis of learning and memory. Two processes in particular, long-term potentiation (LTP) and long-term depression (LTD) are important forms of synaptic plasticity that occur in synapses in the hippocampus, a brain region that is involved in storing memories.
Long-term Potentiation (LTP)
Long-term potentiation (LTP) is a persistent strengthening of a synaptic connection. LTP is based on the Hebbian principle: cells that fire together wire together. There are various mechanisms, none fully understood, behind the synaptic strengthening seen with LTP. One known mechanism involves a type of postsynaptic glutamate receptor, called NMDA (N-Methyl-D-aspartate) receptors, shown in Figure \(\PageIndex{10}\). These receptors are normally blocked by magnesium ions; however, when the postsynaptic neuron is depolarized by multiple presynaptic inputs in quick succession (either from one neuron or multiple neurons), the magnesium ions are forced out allowing Ca ions to pass into the postsynaptic cell. Next, Ca 2+ ions entering the cell initiate a signaling cascade that causes a different type of glutamate receptor, called AMPA (α-amino-3-hydroxy-5-methyl-4-isoxazolepropionic acid) receptors, to be inserted into the postsynaptic membrane, since activated AMPA receptors allow positive ions to enter the cell. So, the next time glutamate is released from the presynaptic membrane, it will have a larger excitatory effect (EPSP) on the postsynaptic cell because the binding of glutamate to these AMPA receptors will allow more positive ions into the cell. The insertion of additional AMPA receptors strengthens the synapse and means that the postsynaptic neuron is more likely to fire in response to presynaptic neurotransmitter release. Some drugs of abuse co-opt the LTP pathway, and this synaptic strengthening can lead to addiction.
Long-term Depression (LTD)
Long-term depression (LTD) is essentially the reverse of LTP: it is a long-term weakening of a synaptic connection. One mechanism known to cause LTD also involves AMPA receptors. In this situation, calcium that enters through NMDA receptors initiates a different signaling cascade, which results in the removal of AMPA receptors from the postsynaptic membrane, as illustrated in Figure \(\PageIndex{10}\). The decrease in AMPA receptors in the membrane makes the postsynaptic neuron less responsive to glutamate released from the presynaptic neuron. While it may seem counterintuitive, LTD may be just as important for learning and memory as LTP. The weakening and pruning of unused synapses allows for unimportant connections to be lost and makes the synapses that have undergone LTP that much stronger by comparison.
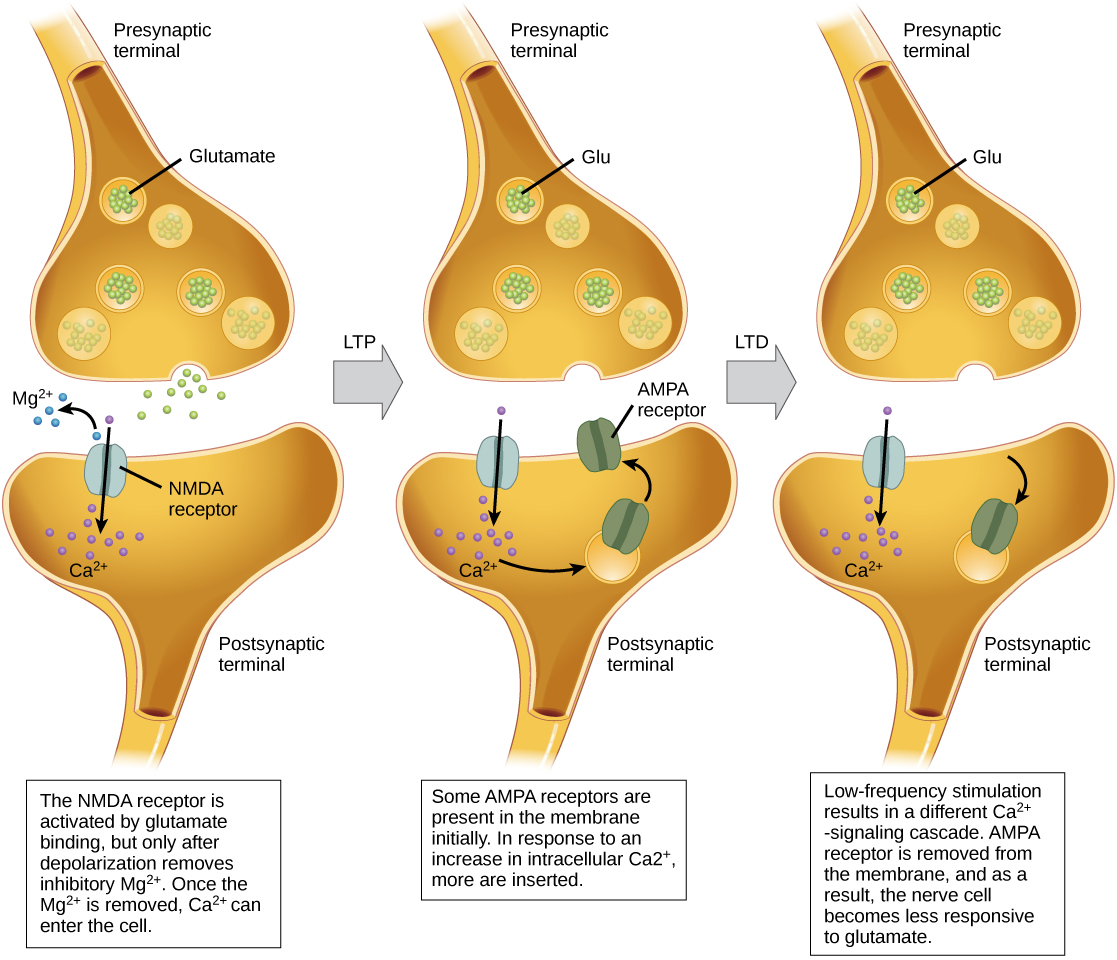
Neurons have charged membranes because there are different concentrations of ions inside and outside of the cell. Voltage-gated ion channels control the movement of ions into and out of a neuron. When a neuronal membrane is depolarized to at least the threshold of excitation, an action potential is fired. The action potential is then propagated along a myelinated axon to the axon terminals. In a chemical synapse, the action potential causes release of neurotransmitter molecules into the synaptic cleft. Through binding to postsynaptic receptors, the neurotransmitter can cause excitatory or inhibitory postsynaptic potentials by depolarizing or hyperpolarizing, respectively, the postsynaptic membrane. In electrical synapses, the action potential is directly communicated to the postsynaptic cell through gap junctions—large channel proteins that connect the pre-and postsynaptic membranes. Synapses are not static structures and can be strengthened and weakened. Two mechanisms of synaptic plasticity are long-term potentiation and long-term depression.
Art Connections
Figure \(\PageIndex{3}\): Potassium channel blockers, such as amiodarone and procainamide, which are used to treat abnormal electrical activity in the heart, called cardiac dysrhythmia, impede the movement of K + through voltage-gated K + channels. Which part of the action potential would you expect potassium channels to affect?
Potassium channel blockers slow the repolarization phase, but have no effect on depolarization.

Want to create or adapt books like this? Learn more about how Pressbooks supports open publishing practices.
8.4 Nerve Impulses
Created by CK-12 Foundation/Adapted by Christine Miller
When Lightning Strikes
This amazing cloud-to-surface lightning occurred when a difference in electrical charge built up in a cloud relative to the ground. When the buildup of charge was great enough, a sudden discharge of electricity occurred. A nerve impulse is similar to a lightning strike. Both a nerve impulse and a lightning strike occur because of differences in electrical charge, and both result in an electric current.
Generating Nerve Impulses
A nerve impulse , like a lightning strike, is an electrical phenomenon. A nerve impulse occurs because of a difference in electrical charge across the plasma membrane of a neuron. How does this difference in electrical charge come about? The answer involves ions , which are electrically-charged atoms or molecules .
Resting Potential
When a neuron is not actively transmitting a nerve impulse, it is in a resting state, ready to transmit a nerve impulse. During the resting state, the sodium-potassium pump maintains a difference in charge across the cell membrane of the neuron. The sodium-potassium pump is a mechanism of active transport that moves sodium ions (Na+) out of cells and potassium ions (K+) into cells. The sodium-potassium pump moves both ions from areas of lower to higher concentration, using energy in ATP and carrier proteins in the cell membrane. The video below, “Sodium Potassium Pump” by Amoeba Sisters, describes in greater detail how the sodium-potassium pump works. Sodium is the principal ion in the fluid outside of cells, and potassium is the principal ion in the fluid inside of cells. These differences in concentration create an electrical gradient across the cell membrane, called resting potential . Tightly controlling membrane resting potential is critical for the transmission of nerve impulses.
Sodium Potassium Pump, Amoeba Sisters, 2020.
Action Potential
A nerve impulse is a sudden reversal of the electrical gradient across the plasma membrane of a resting neuron. The reversal of charge is called an action potential . It begins when the neuron receives a chemical signal from another cell or some other type of stimulus . If the stimulus is strong enough to reach threshold , an action potential will take place is a cascade along the axon.
This reversal of charges ripples down the axon of the neuron very rapidly as an electric current, which is illustrated in the diagram below (Figure 8.4.2). A nerve impulse is an all-or-nothing response depending on if the stimulus input was strong enough to reach threshold. If a neuron responds at all, it responds completely. A greater stimulation does not produce a stronger impulse.
In neurons with a myelin sheath on their axon, ions flow across the membrane only at the nodes between sections of myelin. As a result, the action potential appears to jump along the axon membrane from node to node, rather than spreading smoothly along the entire membrane. This increases the speed at which the action potential travels.
Transmitting Nerve Impulses
The place where an axon terminal meets another cell is called a synapse . This is where the transmission of a nerve impulse to another cell occurs. The cell that sends the nerve impulse is called the presynaptic cell , and the cell that receives the nerve impulse is called the postsynaptic cell .
Some synapses are purely electrical and make direct electrical connections between neurons. Most synapses, however, are chemical synapses. Transmission of nerve impulses across chemical synapses is more complex.
Chemical Synapses
At a chemical synapse, both the presynaptic and postsynaptic areas of the cells are full of molecular machinery that is involved in the transmission of nerve impulses. As shown in Figure 8.4.3, the presynaptic area contains many tiny spherical vessels called synaptic vesicles that are packed with chemicals called neurotransmitters . When an action potential reaches the axon terminal of the presynaptic cell, it opens channels that allow calcium to enter the terminal. Calcium causes synaptic vesicles to fuse with the membrane, releasing their contents into the narrow space between the presynaptic and postsynaptic membranes. This area is called the synaptic cleft . The neurotransmitter molecules travel across the synaptic cleft and bind to receptors , which are proteins embedded in the membrane of the postsynaptic cell.
Neurotransmitters and Receptors
There are more than a hundred known neurotransmitters, and more than one type of neurotransmitter may be released at a given synapse by a presynaptic cell. For example, it is common for a faster-acting neurotransmitter to be released, along with a slower-acting neurotransmitter. Many neurotransmitters also have multiple types of receptors to which they can bind. Receptors, in turn, can be divided into two general groups: chemically gated ion channels and second messenger systems.
- When a chemically gated ion channel is activated, it forms a passage that allows specific types of ions to flow across the cell membrane. Depending on the type of ion, the effect on the target cell may be excitatory or inhibitory .
- When a second messenger system is activated, it starts a cascade of molecular interactions inside the target cell. This may ultimately produce a wide variety of complex effects, such as increasing or decreasing the sensitivity of the cell to stimuli, or even altering gene transcription.
The effect of a neurotransmitter on a postsynaptic cell depends mainly on the type of receptors that it activates, making it possible for a particular neurotransmitter to have different effects on various target cells. A neurotransmitter might excite one set of target cells, inhibit others, and have complex modulatory effects on still others, depending on the type of receptors. However, some neurotransmitters have relatively consistent effects on other cells. Consider the two most widely used neurotransmitters, glutamate and GABA (gamma-aminobutyric acid). Glutamate receptors are either excitatory or modulatory in their effects, whereas GABA receptors are all inhibitory in their effects in adults.
Problems with neurotransmitters or their receptors can cause neurological disorders. The disease myasthenia gravis , for example, is caused by antibodies from the immune system blocking receptors for the neurotransmitter acetylcholine in postsynaptic muscle cells. This inhibits the effects of acetylcholine on muscle contractions, producing symptoms, such as muscle weakness and excessive fatigue during simple activities. Some mental illnesses (including depression ) are caused, at least in part, by imbalances of certain neurotransmitters in the brain. One of the neurotransmitters involved in depression is thought to be serotonin , which normally helps regulate mood, among many other functions. Some antidepressant drugs are thought to help alleviate depression in many patients by normalizing the activity of serotonin in the brain.
8.4 Summary
- A nerve impulse is an electrical phenomenon that occurs because of a difference in electrical charge across the plasma membrane of a neuron.
- The sodium-potassium pump maintains an electrical gradient across the plasma membrane of a neuron when it is not actively transmitting a nerve impulse. This gradient is called the resting potential of the neuron.
- An action potential is a sudden reversal of the electrical gradient across the plasma membrane of a resting neuron. It begins when the neuron receives a chemical signal from another cell or some other type of stimulus. The action potential travels rapidly down the neuron’s axon as an electric current and occurs in three stages: Depolarization, Repolarization and Recovery.
- A nerve impulse is transmitted to another cell at either an electrical or a chemical synapse . At a chemical synapse, neurotransmitter chemicals are released from the presynaptic cell into the synaptic cleft between cells. The chemicals travel across the cleft to the postsynaptic cell and bind to receptors embedded in its membrane.
- There are many different types of neurotransmitters. Their effects on the postsynaptic cell generally depend on the type of receptor they bind to. The effects may be excitatory, inhibitory, or modulatory in more complex ways. Both physical and mental disorders may occur if there are problems with neurotransmitters or their receptors.
8.4 Review Questions
- Define nerve impulse.
- What is the resting potential of a neuron, and how is it maintained?
- Explain how and why an action potential occurs.
- Outline how a signal is transmitted from a presynaptic cell to a postsynaptic cell at a chemical synapse.
- What generally determines the effects of a neurotransmitter on a postsynaptic cell?
- Identify three general types of effects that neurotransmitters may have on postsynaptic cells.
- Explain how an electrical signal in a presynaptic neuron causes the transmission of a chemical signal at the synapse.
- The flow of which type of ion into a neuron results in an action potential? How do these ions get into the cell? What does this flow of ions do to the relative charge inside the neuron compared to the outside?
- Name three neurotransmitters.
8.4 Explore More
Action Potentials, Teacher’s Pet, 2018.
TED Ed| What is depression? – Helen M. Farrell, Parta Learning, 2017.
5 Weird Involuntary Behaviors Explained!, It’s Okay To Be Smart, 2015.
Attributions
Figure 8.4.1
Lightening/ Purple Lightning, Dee Why by Jeremy Bishop on Unsplash is used under the Unsplash License (https://unsplash.com/license).
Figure 8.4.2
Action Potential by CNX OpenStax, Biology on Wikimedia Commons is used under a CC BY 4.0 (https://creativecommons.org/licenses/by/4.0/deed.en) license.
Figure 8.4.3
Chemical_synapse_schema_cropped by Looie496 created file (adapted from original from US National Institutes of Health, National Institute on Aging) is in the public domain (https://en.wikipedia.org/wiki/Public_domain).
Amoeba Sisters. (2020, January 29). Sodium potassium pump. YouTube. https://www.youtube.com/watch?v=7NY6XdPBhxo&feature=youtu.be
CNX OpenStax. (2016, May 27) Figure 4 The action potential is conducted down the axon as the axon membrane depolarizes, then repolarizes [digital image]. In Open Stax, Biology (Section 35.2). OpenStax CNX. https://cnx.org/contents/[email protected]:cs_Pb-GW@6/How-Neurons-Communicate
It’s Okay To Be Smart. (2015, January 26). 5 Weird involuntary behaviors explained! YouTube. https://www.youtube.com/watch?v=ZE8sRMZ5BCA&feature=youtu.be
Mayo Clinic Staff. (n.d.). Depression (major depressive disorder) [online article]. MayoClinic.org. https://www.mayoclinic.org/diseases-conditions/depression/symptoms-causes/syc-20356007
Mayo Clinic Staff. (n.d.). Myasthenia gravis [online article]. MayoClinic.org. https://www.mayoclinic.org/diseases-conditions/myasthenia-gravis/symptoms-causes/syc-20352036
National Institute on Aging. (2006, April 8). Alzheimers disease: Unraveling the mystery. National Institutes of Health. https://www.nia.nih.gov/ ( archived version )
Parta Learning. (2017, December 8). TED Ed| What is depression? – Helen M. Farrell. YouTube. https://www.youtube.com/watch?v=rBcU_apy0h8&t=291s
Teacher’s Pet. (2018, August 26). Action potentials. YouTube. https://www.youtube.com/watch?v=FEHNIELPb0s&feature=youtu.be
A signal transmitted along a nerve fiber.
An atom or molecule with a net electric charge due to the loss or gain of one or more electrons.
The smallest particle of an element that still has the properties of that element.
A molecule is an electrically neutral group of two or more atoms held together by chemical bonds.
A functional unit of the nervous system that transmits nerve impulses; also called a nerve cell.
A solute pump that pumps potassium into cells while pumping sodium out of cells, both against their concentration gradients. This pumping is active and occurs at the ratio of 2 potassium for every 3 calcium.
The semipermeable membrane surrounding the cytoplasm of a cell.
The movement of ions or molecules across a cell membrane into a region of higher concentration, assisted by enzymes and requiring energy.
A complex organic chemical that provides energy to drive many processes in living cells, e.g. muscle contraction, nerve impulse propagation, and chemical synthesis. Found in all forms of life, ATP is often referred to as the "molecular unit of currency" of intracellular energy transfer.
The difference in electrical charge across the plasma membrane of a neuron that is not actively transmitting a nerve impulse.
Reversal of electrical charge across the membrane of a resting neuron that travels down the axon of the neuron as a nerve impulse.
Something that triggers a behavior or other response.
The critical level to which a membrane potential must be depolarized to initiate an action potential.
The place where the axon terminal of a neuron transmits a chemical or electrical signal to another cell.
The cell that sends the nerve impulse.
The cell that receives the nerve impulse.
These membrane-bound organelles store various neurotransmitters that are released at the synapse. The release is regulated by a voltage-dependent calcium channel. Vesicles are essential for propagating nerve impulses between neurons and are constantly recreated by the cell.
A type of chemical that transmits signals from the axon of a neuron to another cell across a synapse.
A space that separates two neurons. It forms a junction between two or more neurons and helps nerve impulse pass from one neuron to the other.
A protein on a cell membrane or inside of a cell that binds with a hormone, neurotransmitter, or other chemical signal to produce a response.
A neurotransmitter that will have excitatory effects on the neuron, meaning it will increase the likelihood that a neuron will fire an action potential.
A neurotransmitter that decreases the likelihood that a neuron will fire an action potential.
A chemical that nerve cells use to send signals to other cells. It is by a wide margin the most abundant excitatory neurotransmitter in the vertebrate nervous system.
A naturally occurring amino acid that works as a neurotransmitter in your brain. Neurotransmitters function as chemical messengers. GABA is considered an inhibitory neurotransmitter because it blocks, or inhibits, certain brain signals and decreases activity in your nervous system.
An antibody, also known as an immunoglobulin, is a large, Y-shaped protein produced mainly by plasma cells that is used by the immune system to neutralize pathogens such as pathogenic bacteria and viruses.
An organic chemical that functions in the brain and body of many types of animals (and humans) as a neurotransmitter—a chemical message released by nerve cells to send signals to other cells, such as neurons, muscle cells and gland cells.
A neurotransmitter. It has a popular image as a contributor to feelings of well-being and happiness, though its actual biological function is complex and multifaceted, modulating cognition, reward, learning, memory, and numerous physiological processes such as vomiting and vasoconstriction.
Human Biology Copyright © 2020 by Christine Miller is licensed under a Creative Commons Attribution-NonCommercial 4.0 International License , except where otherwise noted.
Share This Book
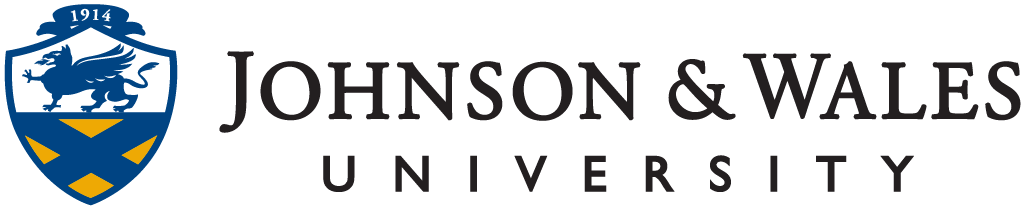
Want to create or adapt books like this? Learn more about how Pressbooks supports open publishing practices.
80 8.4 Nerve Impulses
Created by CK-12 Foundation/Adapted by Christine Miller
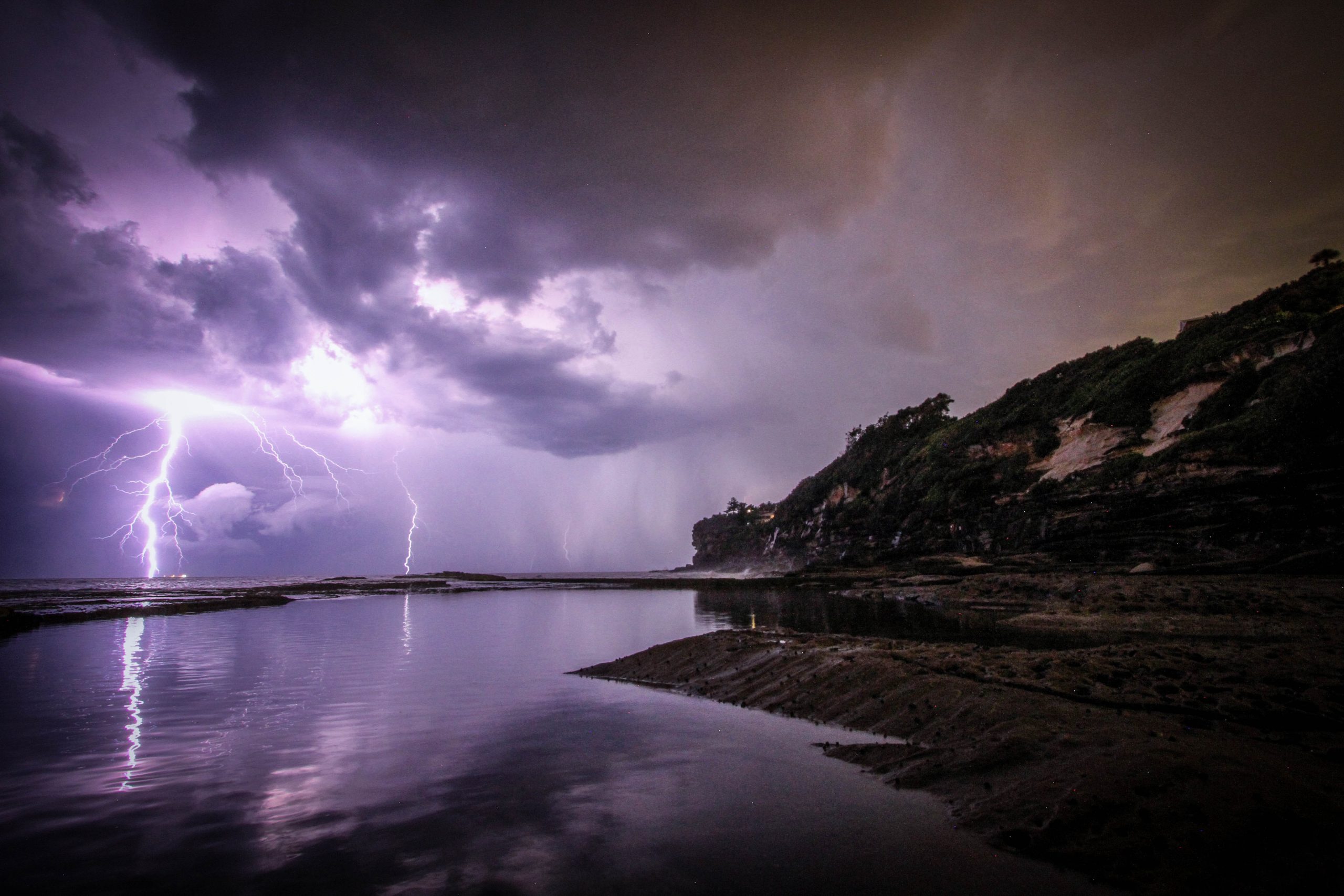
When Lightning Strikes
This amazing cloud-to-surface lightning occurred when a difference in electrical charge built up in a cloud relative to the ground. When the buildup of charge was great enough, a sudden discharge of electricity occurred. A nerve impulse is similar to a lightning strike. Both a nerve impulse and a lightning strike occur because of differences in electrical charge, and both result in an electric current.
Generating Nerve Impulses
A nerve impulse , like a lightning strike, is an electrical phenomenon. A nerve impulse occurs because of a difference in electrical charge across the plasma membrane of a neuron. How does this difference in electrical charge come about? The answer involves ions , which are electrically-charged atoms or molecules .

Resting Potential
When a neuron is not actively transmitting a nerve impulse, it is in a resting state, ready to transmit a nerve impulse. During the resting state, the sodium-potassium pump maintains a difference in charge across the cell membrane of the neuron. The sodium-potassium pump is a mechanism of active transport that moves sodium ions (Na+) out of cells and potassium ions (K+) into cells. The sodium-potassium pump moves both ions from areas of lower to higher concentration, using energy in ATP and carrier proteins in the cell membrane. The video below, “Sodium Potassium Pump” by Amoeba Sisters, describes in greater detail how the sodium-potassium pump works. Sodium is the principal ion in the fluid outside of cells, and potassium is the principal ion in the fluid inside of cells. These differences in concentration create an electrical gradient across the cell membrane, called resting potential . Tightly controlling membrane resting potential is critical for the transmission of nerve impulses.
Sodium Potassium Pump, Amoeba Sisters, 2020.
Action Potential
A nerve impulse is a sudden reversal of the electrical gradient across the plasma membrane of a resting neuron. The reversal of charge is called an action potential . It begins when the neuron receives a chemical signal from another cell or some other type of stimulus . If the stimulus is strong enough to reach threshold , an action potential will take place is a cascade along the axon.
This reversal of charges ripples down the axon of the neuron very rapidly as an electric current, which is illustrated in the diagram below (Figure 8.4.2). A nerve impulse is an all-or-nothing response depending on if the stimulus input was strong enough to reach threshold. If a neuron responds at all, it responds completely. A greater stimulation does not produce a stronger impulse.
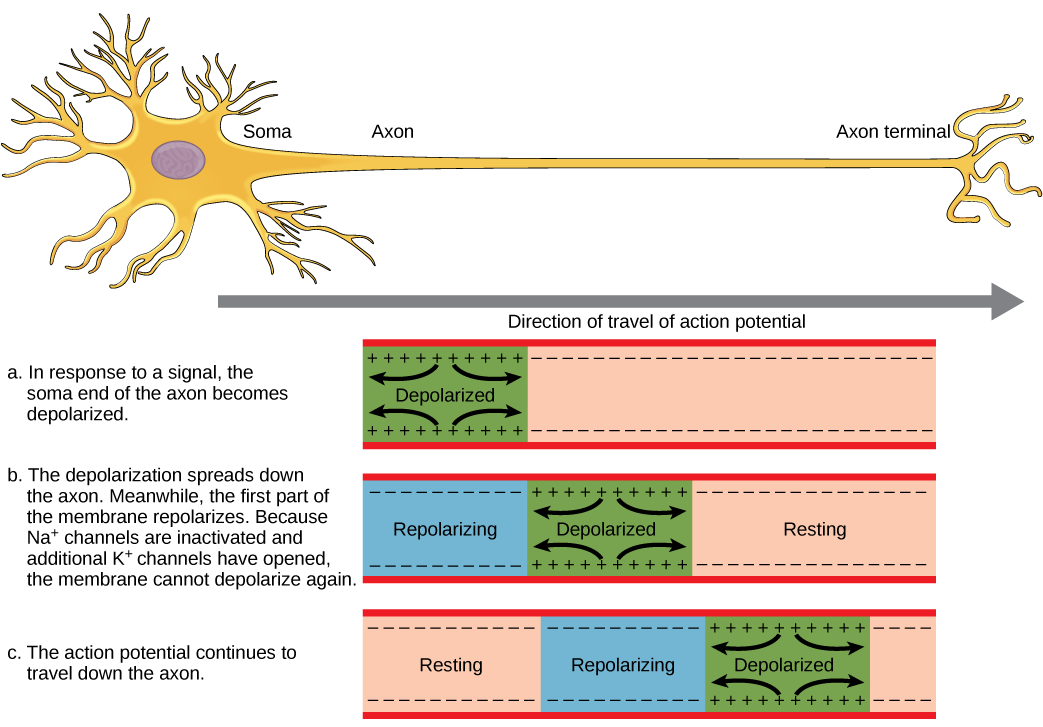
In neurons with a myelin sheath on their axon, ions flow across the membrane only at the nodes between sections of myelin. As a result, the action potential appears to jump along the axon membrane from node to node, rather than spreading smoothly along the entire membrane. This increases the speed at which the action potential travels.
Transmitting Nerve Impulses
The place where an axon terminal meets another cell is called a synapse . This is where the transmission of a nerve impulse to another cell occurs. The cell that sends the nerve impulse is called the presynaptic cell , and the cell that receives the nerve impulse is called the postsynaptic cell .
Some synapses are purely electrical and make direct electrical connections between neurons. Most synapses, however, are chemical synapses. Transmission of nerve impulses across chemical synapses is more complex.
Chemical Synapses
At a chemical synapse, both the presynaptic and postsynaptic areas of the cells are full of molecular machinery that is involved in the transmission of nerve impulses. As shown in Figure 8.4.3, the presynaptic area contains many tiny spherical vessels called synaptic vesicles that are packed with chemicals called neurotransmitters . When an action potential reaches the axon terminal of the presynaptic cell, it opens channels that allow calcium to enter the terminal. Calcium causes synaptic vesicles to fuse with the membrane, releasing their contents into the narrow space between the presynaptic and postsynaptic membranes. This area is called the synaptic cleft . The neurotransmitter molecules travel across the synaptic cleft and bind to receptors , which are proteins embedded in the membrane of the postsynaptic cell.
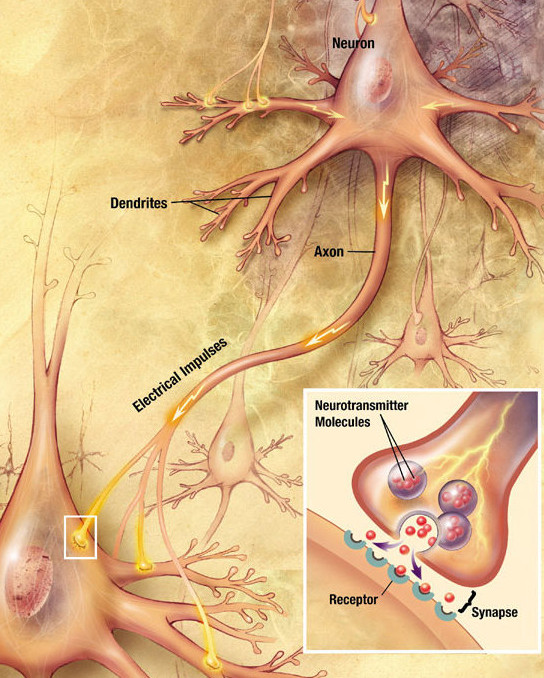
Neurotransmitters and Receptors
There are more than a hundred known neurotransmitters, and more than one type of neurotransmitter may be released at a given synapse by a presynaptic cell. For example, it is common for a faster-acting neurotransmitter to be released, along with a slower-acting neurotransmitter. Many neurotransmitters also have multiple types of receptors to which they can bind. Receptors, in turn, can be divided into two general groups: chemically gated ion channels and second messenger systems.
- When a chemically gated ion channel is activated, it forms a passage that allows specific types of ions to flow across the cell membrane. Depending on the type of ion, the effect on the target cell may be excitatory or inhibitory .
- When a second messenger system is activated, it starts a cascade of molecular interactions inside the target cell. This may ultimately produce a wide variety of complex effects, such as increasing or decreasing the sensitivity of the cell to stimuli, or even altering gene transcription.
The effect of a neurotransmitter on a postsynaptic cell depends mainly on the type of receptors that it activates, making it possible for a particular neurotransmitter to have different effects on various target cells. A neurotransmitter might excite one set of target cells, inhibit others, and have complex modulatory effects on still others, depending on the type of receptors. However, some neurotransmitters have relatively consistent effects on other cells. Consider the two most widely used neurotransmitters, glutamate and GABA (gamma-aminobutyric acid). Glutamate receptors are either excitatory or modulatory in their effects, whereas GABA receptors are all inhibitory in their effects in adults.
Problems with neurotransmitters or their receptors can cause neurological disorders. The disease myasthenia gravis , for example, is caused by antibodies from the immune system blocking receptors for the neurotransmitter acetylcholine in postsynaptic muscle cells. This inhibits the effects of acetylcholine on muscle contractions, producing symptoms, such as muscle weakness and excessive fatigue during simple activities. Some mental illnesses (including depression ) are caused, at least in part, by imbalances of certain neurotransmitters in the brain. One of the neurotransmitters involved in depression is thought to be serotonin , which normally helps regulate mood, among many other functions. Some antidepressant drugs are thought to help alleviate depression in many patients by normalizing the activity of serotonin in the brain.
8.4 Summary
- A nerve impulse is an electrical phenomenon that occurs because of a difference in electrical charge across the plasma membrane of a neuron.
- The sodium-potassium pump maintains an electrical gradient across the plasma membrane of a neuron when it is not actively transmitting a nerve impulse. This gradient is called the resting potential of the neuron.
- An action potential is a sudden reversal of the electrical gradient across the plasma membrane of a resting neuron. It begins when the neuron receives a chemical signal from another cell or some other type of stimulus. The action potential travels rapidly down the neuron’s axon as an electric current and occurs in three stages: Depolarization, Repolarization and Recovery.
- A nerve impulse is transmitted to another cell at either an electrical or a chemical synapse . At a chemical synapse, neurotransmitter chemicals are released from the presynaptic cell into the synaptic cleft between cells. The chemicals travel across the cleft to the postsynaptic cell and bind to receptors embedded in its membrane.
- There are many different types of neurotransmitters. Their effects on the postsynaptic cell generally depend on the type of receptor they bind to. The effects may be excitatory, inhibitory, or modulatory in more complex ways. Both physical and mental disorders may occur if there are problems with neurotransmitters or their receptors.
8.4 Review Questions
- Define nerve impulse.
- What is the resting potential of a neuron, and how is it maintained?
- Explain how and why an action potential occurs.
- Outline how a signal is transmitted from a presynaptic cell to a postsynaptic cell at a chemical synapse.
- What generally determines the effects of a neurotransmitter on a postsynaptic cell?
- Identify three general types of effects that neurotransmitters may have on postsynaptic cells.
- Explain how an electrical signal in a presynaptic neuron causes the transmission of a chemical signal at the synapse.
- The flow of which type of ion into a neuron results in an action potential? How do these ions get into the cell? What does this flow of ions do to the relative charge inside the neuron compared to the outside?
- Name three neurotransmitters.
8.4 Explore More
Action Potentials, Teacher’s Pet, 2018.
TED Ed| What is depression? – Helen M. Farrell, Parta Learning, 2017.
5 Weird Involuntary Behaviors Explained!, It’s Okay To Be Smart, 2015.
Attributions
Figure 8.4.1
Lightening/ Purple Lightning, Dee Why by Jeremy Bishop on Unsplash is used under the Unsplash License (https://unsplash.com/license).
Figure 8.4.2
Action Potential by CNX OpenStax, Biology on Wikimedia Commons is used under a CC BY 4.0 (https://creativecommons.org/licenses/by/4.0/deed.en) license.
Figure 8.4.3
Chemical_synapse_schema_cropped by Looie496 created file (adapted from original from US National Institutes of Health, National Institute on Aging) is in the public domain (https://en.wikipedia.org/wiki/Public_domain).
Amoeba Sisters. (2020, January 29). Sodium potassium pump. YouTube. https://www.youtube.com/watch?v=7NY6XdPBhxo&feature=youtu.be
CNX OpenStax. (2016, May 27) Figure 4 The action potential is conducted down the axon as the axon membrane depolarizes, then repolarizes [digital image]. In Open Stax, Biology (Section 35.2). OpenStax CNX. https://cnx.org/contents/[email protected]:cs_Pb-GW@6/How-Neurons-Communicate
It’s Okay To Be Smart. (2015, January 26). 5 Weird involuntary behaviors explained! YouTube. https://www.youtube.com/watch?v=ZE8sRMZ5BCA&feature=youtu.be
Mayo Clinic Staff. (n.d.). Depression (major depressive disorder) [online article]. MayoClinic.org. https://www.mayoclinic.org/diseases-conditions/depression/symptoms-causes/syc-20356007
Mayo Clinic Staff. (n.d.). Myasthenia gravis [online article]. MayoClinic.org. https://www.mayoclinic.org/diseases-conditions/myasthenia-gravis/symptoms-causes/syc-20352036
National Institute on Aging. (2006, April 8). Alzheimers disease: Unraveling the mystery. National Institutes of Health. https://www.nia.nih.gov/ ( archived version )
Parta Learning. (2017, December 8). TED Ed| What is depression? – Helen M. Farrell. YouTube. https://www.youtube.com/watch?v=rBcU_apy0h8&t=291s
Teacher’s Pet. (2018, August 26). Action potentials. YouTube. https://www.youtube.com/watch?v=FEHNIELPb0s&feature=youtu.be
A signal transmitted along a nerve fiber.
An atom or molecule with a net electric charge due to the loss or gain of one or more electrons.
The smallest particle of an element that still has the properties of that element.
A molecule is an electrically neutral group of two or more atoms held together by chemical bonds.
A functional unit of the nervous system that transmits nerve impulses; also called a nerve cell.
A solute pump that pumps potassium into cells while pumping sodium out of cells, both against their concentration gradients. This pumping is active and occurs at the ratio of 2 potassium for every 3 calcium.
The semipermeable membrane surrounding the cytoplasm of a cell.
The movement of ions or molecules across a cell membrane into a region of higher concentration, assisted by enzymes and requiring energy.
A complex organic chemical that provides energy to drive many processes in living cells, e.g. muscle contraction, nerve impulse propagation, and chemical synthesis. Found in all forms of life, ATP is often referred to as the "molecular unit of currency" of intracellular energy transfer.
The difference in electrical charge across the plasma membrane of a neuron that is not actively transmitting a nerve impulse.
Reversal of electrical charge across the membrane of a resting neuron that travels down the axon of the neuron as a nerve impulse.
Something that triggers a behavior or other response.
The critical level to which a membrane potential must be depolarized to initiate an action potential.
The place where the axon terminal of a neuron transmits a chemical or electrical signal to another cell.
The cell that sends the nerve impulse.
The cell that receives the nerve impulse.
These membrane-bound organelles store various neurotransmitters that are released at the synapse. The release is regulated by a voltage-dependent calcium channel. Vesicles are essential for propagating nerve impulses between neurons and are constantly recreated by the cell.
A type of chemical that transmits signals from the axon of a neuron to another cell across a synapse.
A space that separates two neurons. It forms a junction between two or more neurons and helps nerve impulse pass from one neuron to the other.
A protein on a cell membrane or inside of a cell that binds with a hormone, neurotransmitter, or other chemical signal to produce a response.
A neurotransmitter that will have excitatory effects on the neuron, meaning it will increase the likelihood that a neuron will fire an action potential.
A neurotransmitter that decreases the likelihood that a neuron will fire an action potential.
A chemical that nerve cells use to send signals to other cells. It is by a wide margin the most abundant excitatory neurotransmitter in the vertebrate nervous system.
A naturally occurring amino acid that works as a neurotransmitter in your brain. Neurotransmitters function as chemical messengers. GABA is considered an inhibitory neurotransmitter because it blocks, or inhibits, certain brain signals and decreases activity in your nervous system.
An antibody, also known as an immunoglobulin, is a large, Y-shaped protein produced mainly by plasma cells that is used by the immune system to neutralize pathogens such as pathogenic bacteria and viruses.
An organic chemical that functions in the brain and body of many types of animals (and humans) as a neurotransmitter—a chemical message released by nerve cells to send signals to other cells, such as neurons, muscle cells and gland cells.
A neurotransmitter. It has a popular image as a contributor to feelings of well-being and happiness, though its actual biological function is complex and multifaceted, modulating cognition, reward, learning, memory, and numerous physiological processes such as vomiting and vasoconstriction.
Human Biology Copyright © 2020 by Christine Miller is licensed under a Creative Commons Attribution-NonCommercial 4.0 International License , except where otherwise noted.
Share This Book
A Level Biology
Instant Access to A Level Biology Revision
Sign up now to get access to the entire library of a level biology resources for all exam boards.
If you're ready to pass your A-Level Biology exams, become a member now to get complete access to our entire library of revision materials.
Join over 22,000 learners who have passed their exams thanks to us!
Sign up below to get instant access!
Or try a sample...
Not ready to purchase the revision kit yet? No problem. If you want to see what we offer before purchasing, we have a free membership with sample revision materials.
Signup as a free member below and you'll be brought back to this page to try the sample materials before you buy.
Nerve Impulse
Introduction, continuous conduction , saltatory conduction , resting membrane potential, action potential , polarization , depolarization, repolarization , refractory period , electrical synapses , chemical synapses , cns and nerve impulse, myelin sheath, axon diameter, temperature, what is a nerve impulse, how is a nerve impulse produced, what is the refractory period, what are saltatory impulses.
Nerve impulse was discovered by British Scientist Lord Adrian in the 1930s. Owning to the importance of this discovery, he was awarded Noble Prize in 1932. Nerve Impulse is a major mode of signal transmission for the Nervous system. Neurons sense the changes in the environment and as a result, generate nerve impulses to prepare the body against those changes.
A nerve Impulse is defined as a wave of electrical chemical changes across the neuron that helps in the generation of the action potential in response to the stimulus. This transmission of a nerve impulse across the neuron membrane as a result of a change in membrane potential is known as Nerve impulse conduction.
It is a change in the resting state of the neuron. Due to nerve impulses, the resting potential is changed to an action potential to conduct signals to the target in response to a stimulus. The stimulus can be a chemical, electrical, or mechanical signal.
The action potential is a result of the movement of ions in and out of the cell. Particularly the ions included in this process are sodium and potassium ions. These ions are propagated inside and outside the cell through specific sodium and potassium pumps present in the neuron membrane. The transmission of a nerve impulse from one neuron to another neuron is achieved by a synaptic connection (synapse) between them. It is thus a mode of communication between different cells.
The speed of nerve impulse propagation varies in different types of cells. The rate of transmission and generation of nerve impulses depends upon the type of cell. Besides, Myelin Sheath also helps in accelerating the rate of signal conduction (about 20 times). Generally, the speed of nerve impulses is 0.1-100 m/s.
Mechanism of Nerve Impulse Conduction
Nerve impulse conduction is a major process occurring in the body responsible for organized functions of the body. So, for the conduction of nerve impulses, there are two mechanisms:
- Continuous conduction
- Saltatory conduction
Continuous nerve impulse conduction occurs in non-myelinated axons. The action potential travels along the entire length of the axon. Hence, more time is taken in generating and then transmit nerve impulses during an action potential.
Continuous conduction requires more energy to transmit impulses and is a slower process (approximately 0.1 m/s). It delays the process of conducting signals because it uses a higher number of ion channels to alter the resting state of the neuron.
Saltatory is faster than continuous conduction and occurs in myelinated neurons. In myelinated neurons, myelinated sheaths are present. Between these myelinated sheaths, unmyelinated gaps are presently known as the nodes of Ranvier. Nerve impulse propagates by jumping from one node of Ranvier to the next. This makes the process of nerve impulse faster as the nerve impulse does not travel the entire length of the axon ( this happens in the case of continuous conduction). The nerve impulse travels at a speed of 100 m/s in saltatory conduction.
The number of channels utilized in saltatory conduction is less than in continuous conduction due to which delay of nerve impulse does not occur. This mode of nerve impulse transmission utilizes less energy as well.
If you consider the axon as an electrical wire or loop, nerve impulse that travels along the axon as current, and the charged particles ( sodium and potassium ions) as the electron particles then the process can be understood quite easily. As the flow of current in a wire occurs at a specific voltage only, similarly the conduction of nerve impulse occurs when a stimulus has a maximum threshold value of -55 millivolts. This is essential for altering the resting membrane state to action membrane potential.
When the voltage has the required number of electron particles it conducts current. Similarly, in the case of nerve impulse conduction, the neurons of the stimulus must have a threshold value for causing the movement of ions across the length of the axon (for conducting nerve impulse) by opening the voltage-gated ion channels.
Process of transmission of Nerve Impulse
For the transmission of a nerve impulse, the stages are below:
- Polarization
- Repolarization
- Refractory Period
Before going into the details of the process of nerve impulse transmission, let’s first discuss action and resting potential states.
The resting membrane potential refers to the non-excited state of the nerve cell at rest when no nerve impulse is being conducted. The resting membrane potential of the nerve cell is -70 mV. It is a static state and both the sodium and potassium channels are closed during this state maintaining a high concentration of sodium ions outside and high potassium ions concentration inside the cell.
An action potential occurs when the nerve cell is in an excited state while conducting nerve impulses. In this situation, sodium channels open and potassium channels are closed. This results in a huge influx of sodium ions inside the cells which trigger the nerve impulse conduction. The action potential is +40 mV.
Polarization is the situation in which the membrane is electrically charged but non-conductive. It means it doesn’t conduct nerve impulses in this state. During polarization, the membrane is in a resting potential state. The concentration of sodium ions is about 16 times more outside the axon than inside. In contrast, the concentration of potassium ions is 25 times more inside the axon than outside.
The polarization state is also known as the “Unstimulated or non-conductive state”. Due to the difference in the concentration of ions inside and outside the membrane, a potential gradient is established ranging between -20-200mV ( in the case of humans, the potential gradient in the polarized state is near -70mV). In the polarized state, the axon membrane is more permeable to potassium ions instead of sodium ions and as a result, it causes rapid diffusion of potassium ions.
In the resting state, the membrane potential becomes electro-negatively charged due to the movement of positively charged potassium ions outside the cell and the presence of electro-negative proteins in the intracellular space.
It refers to a graded potential state because a threshold stimulus of about -55mV causes a change in the membrane potential. The threshold stimulus must be strong enough to change the resting membrane potential into action membrane potential.
This results in the alternation in the electro-negativity of the membrane because the stimulus causes the influx of sodium ions (electropositive ions) by 10 times more than in the resting state. For this, sodium voltage-gated channels open. The action potential state is based on the “All or none” method and has two possibilities:
If the stimulus is not more than the threshold value, then there will be no action potential state across the length of the axon.
If the stimulus is more than the threshold value, then it will generate a nerve impulse that will travel across the entire length of the axon.
It is a condition during which the electrical balance is restored inside and outside the axon membrane. Due to the high concentration of sodium ions inside the axoplasm, the potassium channels will open. During the repolarization state, the efflux of potassium ions through the potassium channel occurs. As a result of the opening of potassium voltage-gated channels, sodium voltage-gated channels will be closed. Thus, no sodium ions will move inside the membrane. Therefore, repolarization helps in maintaining or restoring the original membrane potential state.
Until potassium channels close, the number of potassium ions that have moved across the membrane is enough to restore the initial polarized potential state. As a result of this, the membrane becomes hyperpolarized and has a potential difference of -90 mV.
The refractory phase is a brief period after the successful transmission of a nerve impulse. During this period, the membrane prepares itself for the conduction of the second stimulus after restoring the original resting state. It persists for only 2 milliseconds.
During this, the sodium ATPase pump allows the re-establishment of the original distribution of sodium and potassium ions. The sodium and potassium ATPase pump, driven by using ATP, helps to restore the resting membrane state for the conduction of a second nerve impulse in response to the other stimulus. It causes the movement of ions against the concentration gradient. For every two potassium ions that move inside the cell, three sodium ions are transported outside. This process requires ATP because the movement of ions is against the concentration gradient of both ions.
The process of transmission of a nerve impulse from one neuron to the other, after reaching the axon’s synaptic terminal, is known as synapse. This transmission of the nerve impulse by synapses involves the interaction between the axon ending of one neuron (Presynaptic neuron) to the dendrite of another neuron (Postsynaptic neuron). There is space between the pre-synaptic neuron and post-synaptic neuron which is known as synaptic cleft or synaptic gap.
After transmitting from one neuron to another, the nerve impulse generates a particular response after reaching the target site. If somehow the synaptic gap doesn’t allow the passage of nerve impulse, the transmission of nerve impulse will not occur and consequently required response too.
Read more about the Myelin Sheath
Types of synapses
There are two types of synapses:
- Electrical synapses
- Chemical synapses
In electrical synapses, two neurons are connected through channel proteins for transmitting a nerve impulse. The nerve impulse travels across the membrane of the axon in the form of an electrical signal. The signal is transmitted in the form of ions and therefore it is much faster than chemical synapses.
In electrical synapses, the synaptic gap is about 0.2nm which also favours faster nerve impulse conduction.
In chemical synapses, the conduction of nerve impulses occurs through chemical signals. These chemical signals are neurotransmitters. In this type of nerve impulse conduction, the synaptic gap is more than electrical synapses and is about 10-20 nm. Due to this, the transmission of nerve impulses is slower than electrical synapses.
Neurons help in transmitting signals in the form of nerve impulses from the Central nervous system to the peripheral body parts. Neurons are a complex network of fibres that transmit information from the axon ending of one neuron to the dendrite of another neuron. The signal finally reaches the target cell where it shows a response.
In conducting nerve impulses, the following play a major role:
- Axon- Helps in the propagation of nerve impulses to the target cell.
- Dendrites- Receive the signals from the axon ends.
- Axon Ending- Acts as a transmitter of signals.
Axon plays a major role in the process by transmitting signals in the form of nerve impulses via synapses to the target cells. The neuron is responsible for transferring signals to three target cells:
- Another neuron
And this results in the contraction of muscle, and secretion by glands and helps neurons to transmit action potential.
Factors Affecting the Speed of Nerve Impulse
The following are some major factors that affect the speed of nerve impulses:
Myelin sheath is present around the neuron and functions as an electrical insulator. Due to this sheath, an action potential is not formed on the surface of the neuron. This Myelin sheath has regular gaps, where it is not present, called nodes of Ranvier. An action potential can form at these gaps and impulse will jump from node to node by saltatory conduction. This can be a factor in increasing the speed of nerve impulses from about 30-1 m/ to 90-1 m/s.
As the axon diameter increase, the speed of nerve impulses increases as well. This is because a larger axon diminishes the ion leakage out of the axon. This helps in maintaining the membrane potential and thus favours faster nerve impulses.
Temperature cause changes in the rate of diffusion of ions across the neuron membrane. Temperature directly correlates with the transmission of nerve impulses. If the temperature is higher, the rate of diffusion of sodium and potassium ions will be high and the axon will become depolarized quickly which will cause a faster nerve impulse conduction.
A nerve impulse is thus an important signal transduction mode for triggering a response in major body parts due to a strong stimulus. Any distraction in this process can have drastic effects on the body.
Frequently Asked Questions
A nerve impulse is a wave of electrochemical changes that travel across the plasma membrane and helps in the generation of an action potential. Signals are propagated along the nerve fibres in the form of nerve impulses.
A nerve impulse is produced when a stimulus acts on the nerve fibre, resulting in electrochemical changes across the nerve membrane. These electrochemical changes cause depolarization of the membrane resulting in the generation of nerve impulses.
It is a short duration of time during which a new nerve impulse cannot be generated in a neuron, after initiation of a previous action potential. This period occurs at the end of action potential and limits the speed at which nerve impulses can be generated in a nerve fibre.
These are nerve impulses that jump from one node to another and are seen only in myelinated nerve fibres. Saltatory conduction increases the speed at which a nerve signal is conducted down the length of an axon.
- Lodish, H; Berk, A; Kaiser, C; Krieger, M; Bretscher, A; Ploegh, H; Amon, A (2000). Molecular Cell Biology (7th ed.). New York, NY: W. H. Freeman and Company. p. 695.
- Marieb, E. N., & Hoehn, K. (2014). Human anatomy & physiology. San Francisco, CA: Pearson Education Inc.

- Types of Bones
- Axial Skeleton
- Appendicular Skeleton
- Skeletal Pathologies
- Muscle Tissue Types
- Muscle Attachments & Actions
- Muscle Contractions
- Muscular Pathologies
- Functions of Blood
- Blood Vessels
- Circulation
- Circulatory Pathologies
- Respiratory Functions
- Upper Respiratory System
- Lower Respiratory System
- Respiratory Pathologies
- Oral Cavity
- Alimentary Canal
- Accessory Organs
- Absorption & Elimination
- Digestive Pathologies
- Lymphatic Structures
- Immune System
- Urinary System Structures
- Urine Formation
- Urine Storage & Elimination
- Urinary Pathologies
- Female Reproductive System
- Male Reproductive System
- Reproductive Process
- Endocrine Glands
Muscle Contractions: How Neurotransmitters And Chemical Reactions Move Muscles And Bones
Watch Video Summary
© 2024 Visible Body
Quadriceps femoris muscle
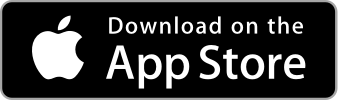
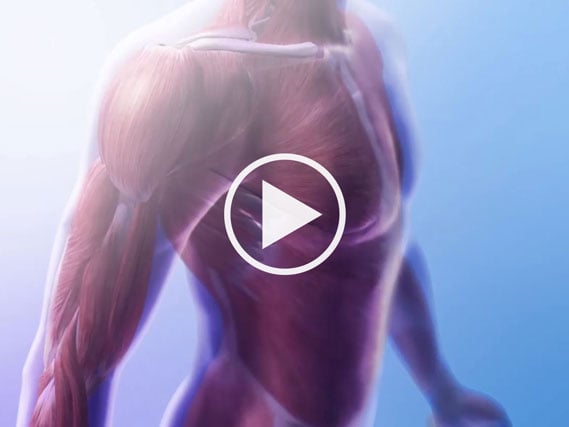
How do the bones of the human skeleton move? Skeletal muscles contract and relax to mechanically move the body. Messages from the nervous system cause these muscle contractions. The whole process is called the mechanism of muscle contraction and it can be summarized in three steps:
(1) A message travels from the nervous system to the muscular system, triggering chemical reactions.
(2) The chemical reactions lead to the muscle fibers reorganizing themselves in a way that shortens the muscle--that’s the contraction.
(3) When the nervous system signal is no longer present, the chemical process reverses, and the muscle fibers rearrange again and the muscle relaxes.
Let’s look a little more closely at the steps in the mechanism of muscle contraction.
1. A Muscle Contraction Is Triggered When an Action Potential Travels Along the Nerves to the Muscles
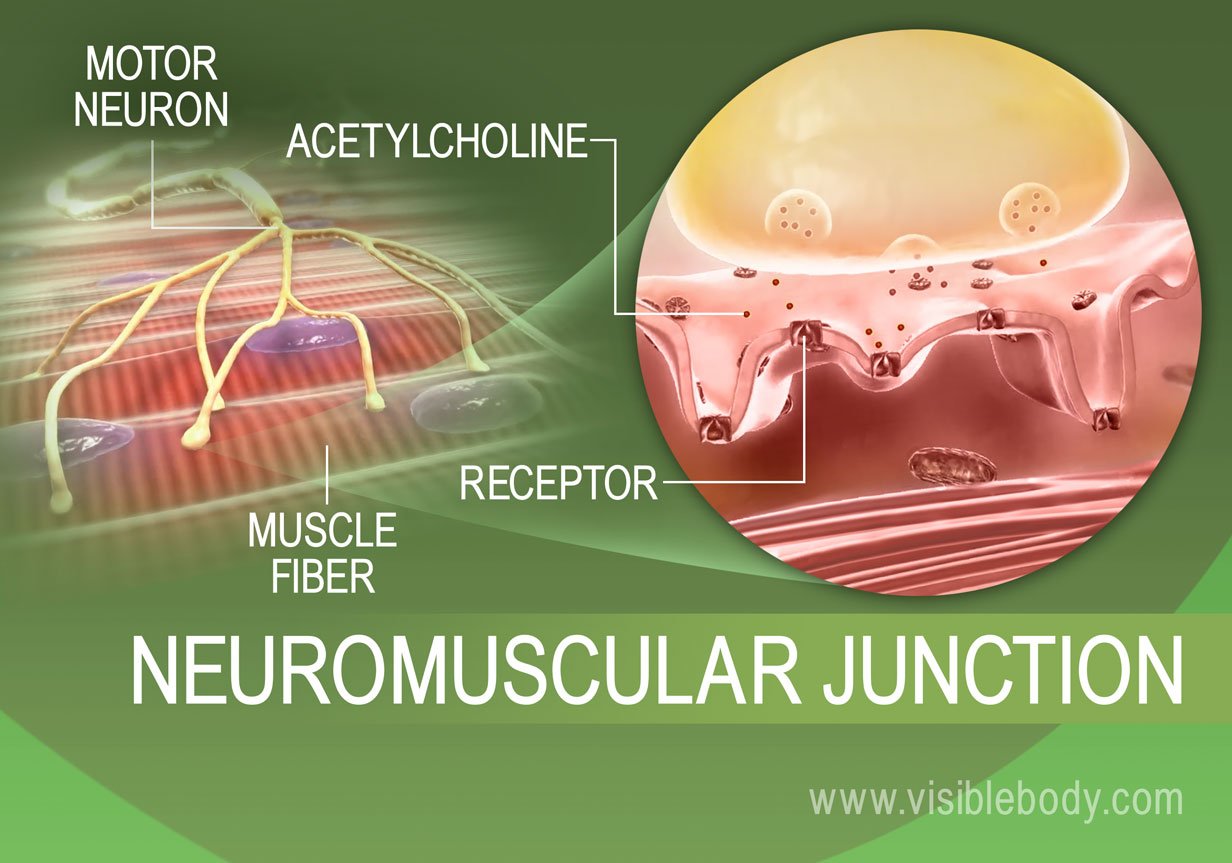
Muscle contraction begins when the nervous system generates a signal. The signal, an impulse called an action potential, travels through a type of nerve cell called a motor neuron. The neuromuscular junction is the name of the place where the motor neuron reaches a muscle cell. Skeletal muscle tissue is composed of cells called muscle fibers. When the nervous system signal reaches the neuromuscular junction a chemical message is released by the motor neuron. The chemical message, a neurotransmitter called acetylcholine, binds to receptors on the outside of the muscle fiber. That starts a chemical reaction within the muscle.
2. Acetylcholine Is Released and Binds to Receptors on the Muscle Membrane
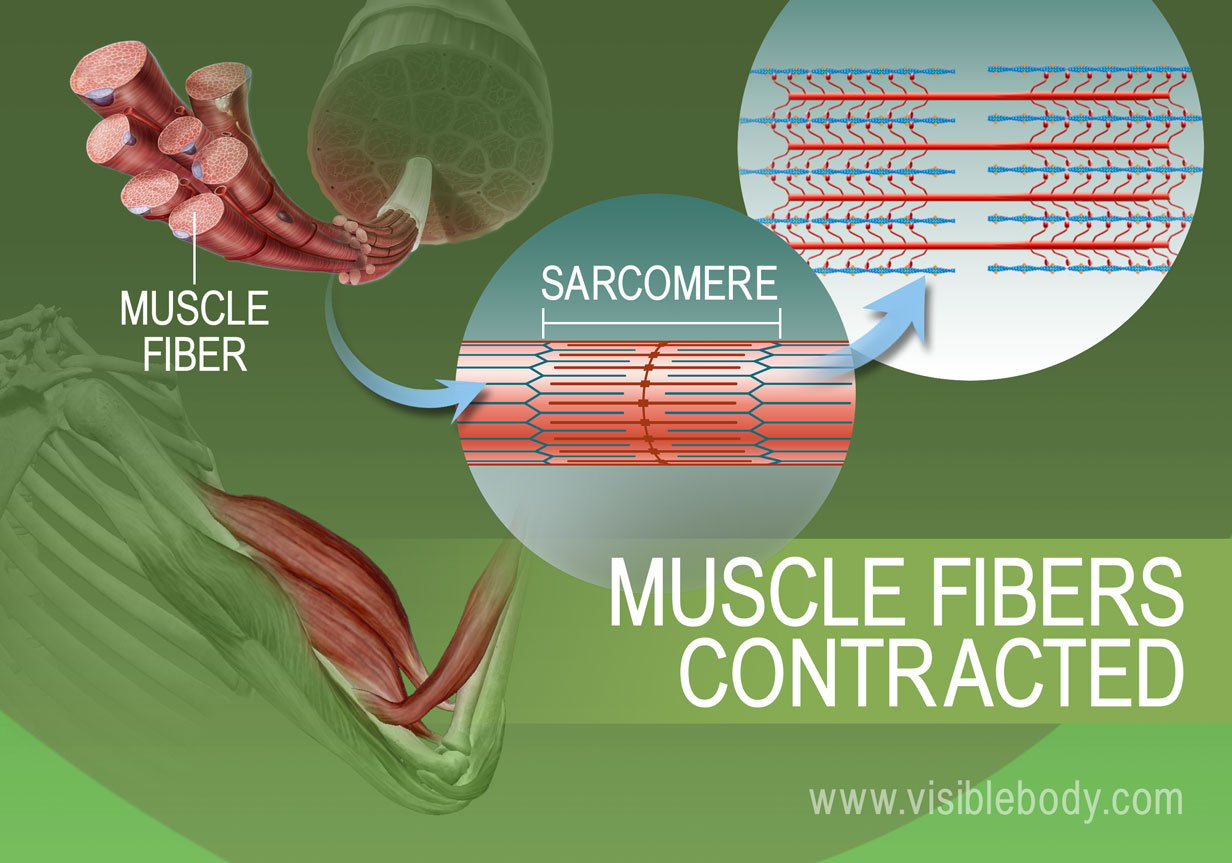
A multistep molecular process within the muscle fiber begins when acetylcholine binds to receptors on the muscle fiber membrane. The proteins inside muscle fibers are organized into long chains that can interact with each other, reorganizing to shorten and relax. When acetylcholine reaches receptors on the membranes of muscle fibers, membrane channels open and the process that contracts a relaxed muscle fibers begins:
- Open channels allow an influx of sodium ions into the cytoplasm of the muscle fiber.
- The sodium influx also sends a message within the muscle fiber to trigger the release of stored calcium ions.
- The calcium ions diffuse into the muscle fiber.
- The relationship between the chains of proteins within the muscle cells changes, leading to the contraction.
3. Muscle Fibers Relax When the Nervous System Signal Is No Longer Present
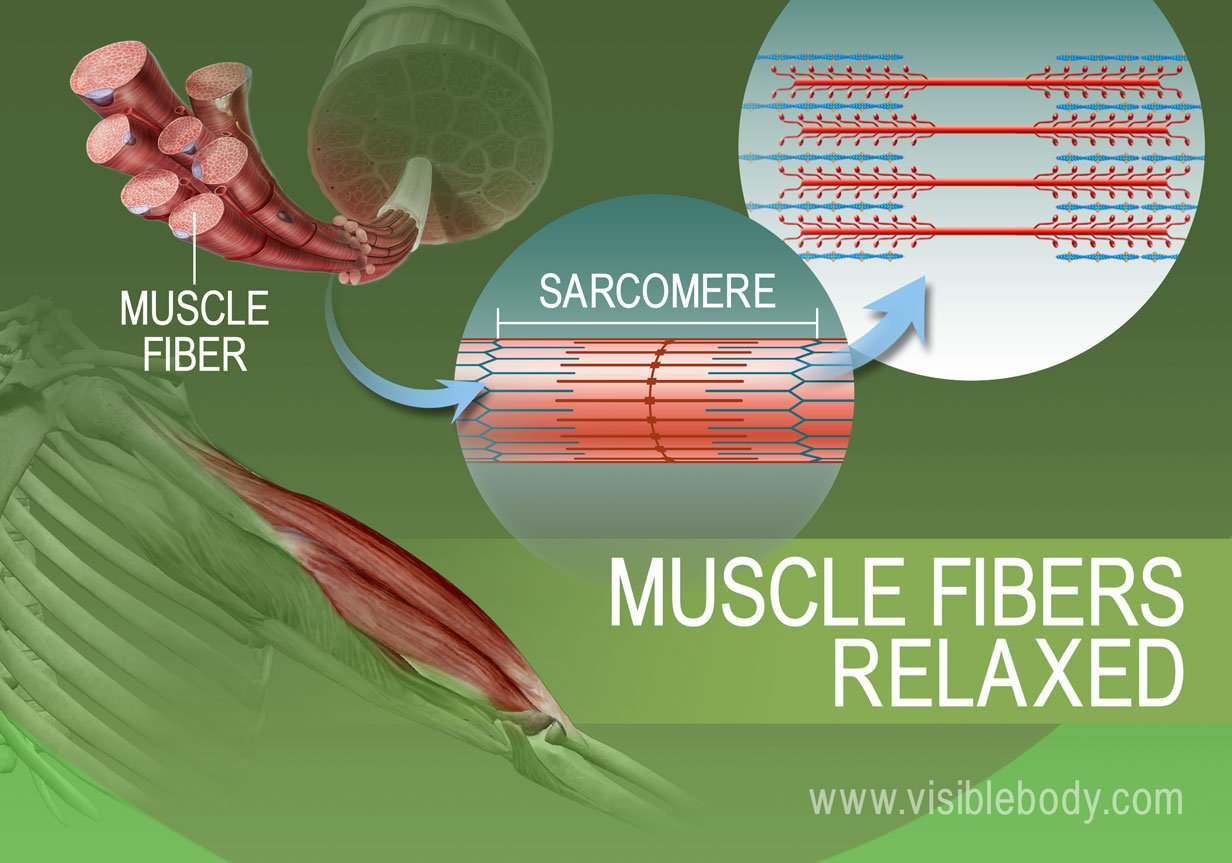
When the stimulation of the motor neuron providing the impulse to the muscle fibers stops, the chemical reaction that causes the rearrangement of the muscle fibers' proteins is stopped. This reverses the chemical processes in the muscle fibers and the muscle relaxes.
External Sources
A video by Armando Hasudungan on YouTube detailing the structure of skeletal muscle.
“ The Sliding Filament Theory of Muscle Contraction ” by Jacob L. Krans, Ph.D. on Scitable.
Visible Body Web Suite provides in-depth coverage of each body system in a guided, visually stunning presentation.
Related Articles
5 Facts about Human Muscles
3 Types of Muscle Tissue
Muscle Attachment and Actions
Muscular System Pathologies
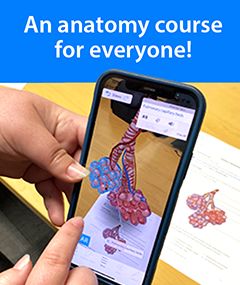
For students
For instructors
Get our awesome anatomy emails!
When you select "Subscribe" you will start receiving our email newsletter. Use the links at the bottom of any email to manage the type of emails you receive or to unsubscribe. See our privacy policy for additional details.
- User Agreement
- Permissions

How does a nerve impulse travel through the body?
The information acquired at the dendritic tip of a nerve cell sets off a chemical reaction that creates an electrical impulse. this impulse travels from the dendrite to the cell body and then along the axon to its end. at the end of the axon the electrical impulse sets off the release of some chemicals. these chemicals cross the gap or synapse and start a similar electrical impulse in a dendrite of the next neuron. a similar synapse finally allows the delivery of such impulse from neurons to other cells such as muscles or glands..

define nerve impulse which structure in a neuron help to conduct a nerve impulse :
i} towards the cell
ii} away from the cell body
What are the scheme of travelling of nerve impulse in the body?

If you're seeing this message, it means we're having trouble loading external resources on our website.
If you're behind a web filter, please make sure that the domains *.kastatic.org and *.kasandbox.org are unblocked.
To log in and use all the features of Khan Academy, please enable JavaScript in your browser.
Biology library
Course: biology library > unit 33, anatomy of a neuron.
- Overview of neuron structure and function
- The membrane potential
- Electrotonic and action potentials
- Saltatory conduction in neurons
- Neuronal synapses (chemical)
- The synapse
- Neurotransmitters and receptors
- Q & A: Neuron depolarization, hyperpolarization, and action potentials
- Overview of the functions of the cerebral cortex

Want to join the conversation?
- Upvote Button navigates to signup page
- Downvote Button navigates to signup page
- Flag Button navigates to signup page

Video transcript

IMAGES
VIDEO
COMMENTS
Figure 42.2.2 42.2. 2: The (a) resting membrane potential is a result of different concentrations of Na + and K + ions inside and outside the cell. A nerve impulse causes Na + to enter the cell, resulting in (b) depolarization. At the peak action potential, K + channels open and the cell becomes (c) hyperpolarized.
The transmission of a nerve impulse along a neuron from one end to the other occurs as a result of electrical changes across the membrane of the neuron. The membrane of an unstimulated neuron is polarized—that is, there is a difference in electrical charge between the outside and inside of the membrane. The inside is negative with respect to ...
A nerve impulse is an all-or-nothing response depending on if the stimulus input was strong enough to reach threshold. If a neuron responds at all, it responds completely. A greater stimulation does not produce a stronger impulse. Figure 8.4.2 An action potential speeds along an axon in milliseconds.
The neuron is the one that transfers the message (nerve impulse) to the brain. In the same way, the cell that senses the stimuli in any part of the body is the receptor, not the neuron. So about taste sensation, the stimuli is actually the chemicals in our mouth. When the pH is low, we feel that it's sour. When the pH is high, we feel that it's ...
Where the nervous system acts quickly (virtually instantly) delivering messages by nerve impulses, the endocrine system has a slower but longer lasting response which compliments the nervous system. The endocrine system regulates growth, development and reproduction and augments the body's capacity for handling physical and psychological stress.
A nerve impulse is initiated by a stimulus, that is, a change in the internal or external environment. This stimulus triggers a receptor to send a nerve impulse to our central nervous system (CNS). The CNS, consisting of the brain and spinal cord, processes the information. Nerve impulses are then transmitted from the CNS to different organs ...
8.4 Summary. A nerve impulse is an electrical phenomenon that occurs because of a difference in electrical charge across the plasma membrane of a neuron. The sodium-potassium pump maintains an electrical gradient across the plasma membrane of a neuron when it is not actively transmitting a nerve impulse. This gradient is called the resting ...
When you want your hand to move, your brain sends signals through your nerves to your hand telling the muscles to contract. But your nerves don't just say "hand, move.". Instead your nerves send lots of electrical impulses (called action potentials) to different muscles in your hand, allowing you to move your hand with extreme precision.
A nerve impulse is the electric signals that pass along the dendrites to generate a nerve impulse or an action potential. An action potential is due to the movement of ions in and out of the cell. It specifically involves sodium and potassium ions. They are moved in and out of the cell through sodium and potassium channels and sodium-potassium ...
Nerve impulse conduction is a major process occurring in the body responsible for organized functions of the body. So, for the conduction of nerve impulses, there are two mechanisms: Continuous conduction; Saltatory conduction; ... This makes the process of nerve impulse faster as the nerve impulse does not travel the entire length of the axon ...
An electrical nerve impulse travels along the axon close axon The long part of a nerve cell along which the electrical signal quickly moves. of the first neuron (presynaptic neuron).; When the ...
Muscle contraction begins when the nervous system generates a signal. The signal, an impulse called an action potential, travels through a type of nerve cell called a motor neuron. The neuromuscular junction is the name of the place where the motor neuron reaches a muscle cell. Skeletal muscle tissue is composed of cells called muscle fibers.
When a nerve sends an electrical impulse: The signal travels down the axon, the "wiring" connection of the nerve. The message converts to a chemical signal at the end of the nerve called the axon hillock. The chemical releases molecules called neurotransmitters, into a space that bridges the space between one neuron to another.
Neurotransmitters are how we communicate between one cell and the next. Synapses between neurons are either excitatory or inhibitory - and that all comes down to the neurotransmitter released. Excitatory neurotransmitters cause the signal to propagate - more action potentials are triggered. Inhibitory signals work to cancel the signal.
Solution. Information is received by the dendrite tip of the nerve cell. Sets off a chemical reaction and creates an electrical impulse. The electrical impulse will travel to the neuron cell body. From the cell body to the ends of the axon through the axon. The electrical impulse causes the release of chemicals by the ends of the axon.
Anatomy is the study of the structure of the body & Physiology is the study of how the body functions as a whole. 2. The function of the nervous system is to integrate and control the other body systems. Explain how the nervous system does this. a. The Nervous system collects the information and sends out signals to certain parts of the body. 3.
How do neurons "talk" to one another? The action happens at the synapse, the point of communication between two neurons or between a neuron and a target cell, like a muscle or a gland.At the synapse, the firing of an action potential in one neuron—the presynaptic, or sending, neuron—causes the transmission of a signal to another neuron—the postsynaptic, or receiving, neuron—making the ...
Similar questions. Q. Nerve impulse travel through synapse with the help of ____. Q. What is synapse how does the nerve impulse cross the synapse. Q. define nerve impulse which structure in a neuron help to conduct a nerve impulse : i} towards the cell. ii} away from the cell body. Q.
The signals then travel to a region called the insula, which acts as a kind of sensor: The fuller the bladder becomes, the more neurons in the insula fire off tiny electrical pulses called action potentials. ... A simplified representation of some of the nerve pathways and brain regions that allow most healthy people to detect when the bladder ...
Anatomy of a neuron. Neurons (or nerve cells) are specialized cells that transmit and receive electrical signals in the body. Neurons are composed of three main parts: dendrites, a cell body, and an axon. Signals are received through the dendrites, travel to the cell body, and continue down the axon until they reach the synapse (the ...