Grade level
- Kindergarten
- English Language Arts
- Holidays Seasonal
- Social Studies History
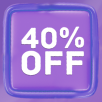

Animal Groups Journeys

Homeschool Curricula, Activities, Centers
Balanced Literacy, Grammar, Reading
- Description
- Reviews (3)
Animal Groups Journeys Lesson 15, Unit 3
There are 100 pages included in this story unit!
I am currently working on all First Grade Journeys and revising all of the the ones that I have previously made. Please always check back and look under “My Purchases”. If there have been any changes, it will let you know to redownload.
Centers, Worksheets and Booklets
**”Long I / Short I” Center~ Directions:Read the sentences.
**Spelling Words Booklet ~ Write 2 times, then write in a complete sentence.
**Roll, Read, and Color (2 pages ~ 6 spelling words on each)
**Color the Sound (Long, Short I)
**Long I interactive notebook **Read, Color, and Write
**Long I Sort Cut and Paste
**Spelling and Vocabulary Flashcards
**-ER and -EST Center
**Venn Diagram (compare and contrast)
**Vocabulary cut and paste
**Vocabulary Words Matching Puzzles (Match definitions) *Vocabulary Booklet ~write the definition for each word.
**Making Words (Animal Groups) **Making Words Worksheet **ABC Order (vocabulary words) ~ Recording Sheet included **ABC Order (spelling words) ~ Recording Sheet included
**2 ABC Order worksheets (1 cut and paste)
**Retelling Booklet (Write 2 sentences about each animal group)
**5 Journal Writing Prompts from the story.
**Daily Proofreading Page
**Not all pages are listed above.

How animal traits have shaped the journey of species across the globe
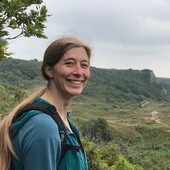
PhD candidate, Swansea University
Disclosure statement
While working on this study, Sarah-Sophie Weil was affiliated with Université Grenoble Alpes (France) and Swansea University (Wales, UK) who supported her through Initiative d’excellence (IDEX) International Strategic Partnership and Swansea University Strategic Partner Research (SUSPR) scholarships.
Swansea University provides funding as a member of The Conversation UK.
View all partners
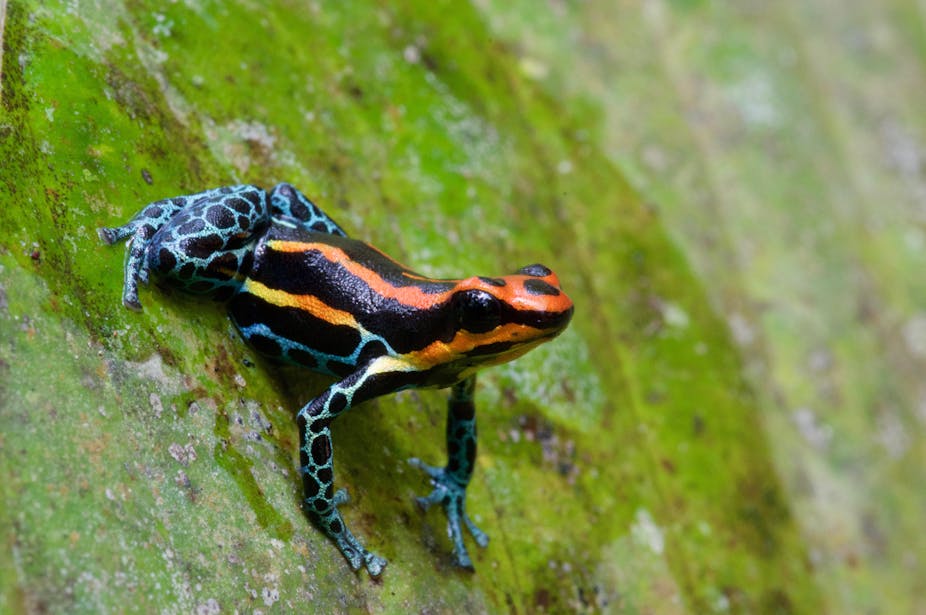
The devastating tsunami that hit Japan in March 2011 set off a series of events which have long fascinated scientists like me. It was so powerful that it caused 5 million tonnes of debris to wash into the Pacific – 1.5 million tonnes remained afloat and started drifting with the currents.
One year later, and half a world away, debris began washing ashore on the west coast of North America. More than 280 Japanese coastal species such as mussels, barnacles and even some species of fish, had hitched a ride on the debris and made an incredible journey across the ocean. These species were still alive and had the potential to establish new populations.
How animals cross major barriers, such as oceans and mountain ranges, to shape Earth’s biodiversity is an intriguing topic. And a new study by my collaborators and I has shed light on this process, revealing how animal characteristics such as body size and life history can influence their spread across the globe.
We know that such dispersal events occur in terrestrial species as well. For instance, at least 15 green iguanas journeyed more than 200km (124 miles) from Guadeloupe to Anguilla in the Caribbean in 1995. They arrived on a mat of logs and trees (likely uprooted through a hurricane), some of which were more than 9 metres (20 feet) long.
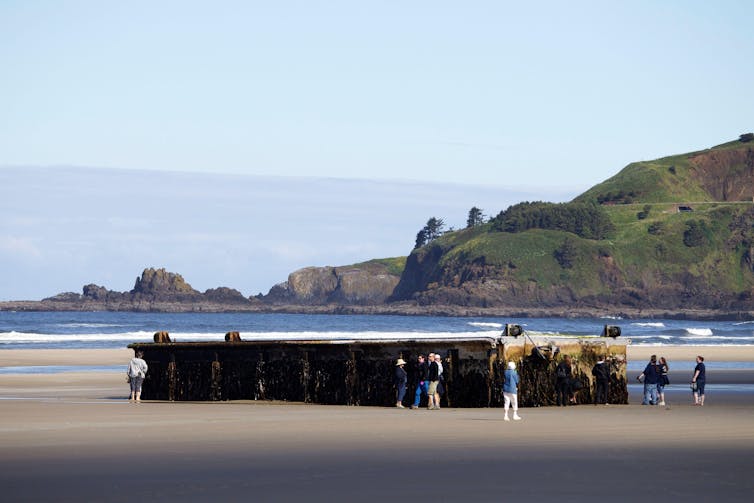
The role of animal characteristics in dispersal
When animals move across major barriers it can have a big impact on both the new and old locations. For example, an invasive species can arrive in a new area and compete with native species for resources. However, those consequences can be even greater over longer periods of time.
The movement of monkeys from Africa to South America around 35 million years ago led to the evolution of more than 90 species of New World monkeys , including tamarins, capuchins and spider monkeys. And a few chameleons rafting on vegetation from Africa to Madagascar is why we find half of all living chameleon species there today.
These events were long thought to be determined by chance – the coincidence of some chameleons sitting on the right tree at the right time. However, some scientists have suggested there might be more to it. They hypothesised there could be more general patterns in the animals that reach their destination successfully, related to certain characteristics.
Could body size affect how far a species can travel? Animals with more fat reserves may be able to travel longer distances. Or could it be how a species reproduces and survives? For example, animals that lay many eggs or mature early may be more likely to establish a new population in a new place.
But despite a vigorous theoretical debate, the options to test these hypotheses were limited because such dispersal events are rare. Also, the right statistical tools were not available until recently.
Thanks to the recent development of new biogeographical models and the great availability of data, we can now try to answer questions about how tetrapod species (amphibians, reptiles, birds and mammals) have moved around the globe over the past 300 million years and whether successful species share any common characteristics.
These models allow us to estimate the movements of species’ ancestors while also considering their characteristics. We used these models to study 7,009 species belonging to 56 groups of tetrapods.
What we found
For 91% of the animal groups we studied, models that included species characteristics were better supported than models that didn’t. This means that body size and life history are closely linked to how successful a species is at moving to and establishing itself in a new location.
Animals with large bodies and fast life histories (breeding early and often, like water voles) generally dispersed more successfully, as expected. However, there were some exceptions to this rule. In some groups, smaller animals or animals with average traits had higher dispersal rates.
For example, small hummingbirds dispersed better than larger ones, and poison dart frogs with intermediate life histories dispersed better than those with very fast or very slow life histories.
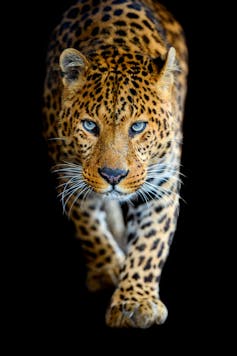
We investigated this variation further and found that the relationship between body size and movement depended on the average size and life history of the group. Our results show that the links between characteristics and dispersal success depend on both body size and life history, and that these cannot be considered separately.
Groups in which small size was an advantage were often already made up of small species (making the dispersal-prone species even smaller), and these species also had fast life histories. We found this to be true for the rodent families Muridae and Cricetidae .
But groups in which dispersers had intermediate body sizes generally had slow life histories (meaning they had low reproductive output but long lifespans). This means the combination of small body size and slow life history is very unlikely to be an advantage for dispersal across major barriers such as oceans.
It’s not just chance
It is amazing to think that rare dispersal events, which can lead to the rise of many new species, are not completely random. Instead, the intrinsic characteristics of species can shape the histories of entire groups of animals, even though chance still may play an important role.
At the same time, two of the most important environmental challenges of our time are related to movement across major barriers: biological invasions and species’ responses to climate change. On a planet facing rapid changes, understanding how animals move across barriers is therefore crucial.
- Biodiversity
- South America
- Environment
- North America
- Pacific Ocean
- Japanese tsunami

Case Management Specialist

Lecturer / Senior Lecturer - Marketing

Assistant Editor - 1 year cadetship

Executive Dean, Faculty of Health

Lecturer/Senior Lecturer, Earth System Science (School of Science)
Journeys animal groups
Examples from our community, 3,755 results for 'journeys animal groups'.
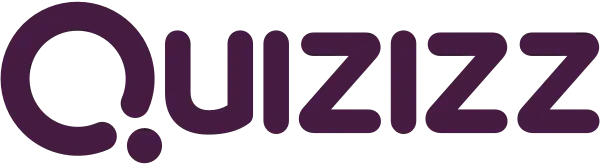
Have an account?

Animal Groups Journeys
7 questions
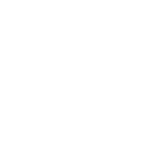
Introducing new Paper mode
No student devices needed. Know more
A fish has a ------- and ------
Reptiles have....
Snakes cannot ........
Frogs and Toads are .......
Birds have.....
We are all......
Name the 5 animal groups
Explore all questions with a free account
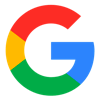
Continue with email
Continue with phone
Subscribe for posts, freebies & updates!
Emily Education
Educational Resources
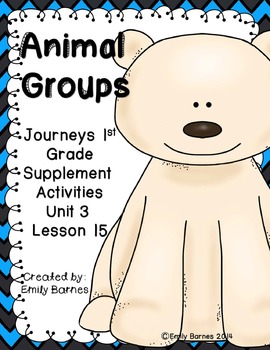
Animal Groups Journeys 1st Grade Supplement Activities Lesson 15
Animal Groups Supplement Activities Journeys 1st Grade Unit 3 Lesson 15
Product Reviews
Average rating: 0 based on 0 reviews.
We’d love to help you with any questions about our products. Please contact us.
Latest on Instagram
Latest on facebook.
8 months ago

Share on Facebook Share on Twitter Share on Linked In Share by Email
9 months ago
1 years ago

An official website of the United States government
The .gov means it’s official. Federal government websites often end in .gov or .mil. Before sharing sensitive information, make sure you’re on a federal government site.
The site is secure. The https:// ensures that you are connecting to the official website and that any information you provide is encrypted and transmitted securely.
- Publications
- Account settings
Preview improvements coming to the PMC website in October 2024. Learn More or Try it out now .
- Advanced Search
- Journal List
- Scientific Reports

The scaling of social interactions across animal species
Luis e. c. rocha.
1 Department of Economics, Ghent University, Ghent, Belgium
2 Department of Physics and Astronomy, Ghent University, Ghent, Belgium

Jan Ryckebusch
Koen schoors.
4 Higher School of Economics, National Research University, Moscow, Russia
Matthew Smith
3 The Business School, Edinburgh Napier University, Edinburgh, UK
Associated Data
Social animals self-organise to create groups to increase protection against predators and productivity. One-to-one interactions are the building blocks of these emergent social structures and may correspond to friendship, grooming, communication, among other social relations. These structures should be robust to failures and provide efficient communication to compensate the costs of forming and maintaining the social contacts but the specific purpose of each social interaction regulates the evolution of the respective social networks. We collate 611 animal social networks and show that the number of social contacts E scales with group size N as a super-linear power-law E = C N β for various species of animals, including humans, other mammals and non-mammals. We identify that the power-law exponent β varies according to the social function of the interactions as β = 1 + a / 4 , with a ≈ 1 , 2 , 3 , 4 . By fitting a multi-layer model to our data, we observe that the cost to cross social groups also varies according to social function. Relatively low costs are observed for physical contact, grooming and group membership which lead to small groups with high and constant social clustering. Offline friendship has similar patterns while online friendship shows weak social structures. The intermediate case of spatial proximity (with β = 1.5 and clustering dependency on network size quantitatively similar to friendship) suggests that proximity interactions may be as relevant for the spread of infectious diseases as for social processes like friendship.
Introduction
Social animals including humans live in groups to optimise the multiplicative benefits of social interactions such as protection, coordination, cooperation, access to information, and fitness, while balancing the competition, disease risk, and stress costs of group living 1 – 3 . Social interactions are fundamentally dyadic yet sufficiently diverse to link multiple animals or humans in connected social structures 1 , 4 , 5 . The purpose of social interactions is also diverse and spans a range of processes including communication, trust, grooming, dominance, or simply the loosely defined idea of friendship 1 , 4 , 6 . Correlations between social interactions, as for example dominance and physical contact, friendship ties maintained through communication, or the intertwined relation between trust and spatial proximity, reveal the complexity of social phenomena and suggest that common principles may underlie the formation of social ties.
A fundamental question concerns how the number of social connections depends on group size, and whether there are any emerging patterns in this relationship. The answer may reveal whether interaction patterns become more complex with size in order to maintain efficient social structures within the group. The cost to establish and maintain social contacts in small groups is relatively low but increases in larger groups 7 . This increasing costs leads to peer selection, either by necessity or affinity, up to a species-specific cognitive saturation point in the number of contacts one can manage 8 . Assuming that all members of a social group are reachable via social ties, in the limiting scenarios, a group of size N individuals may have a fragile star-like structure with E = N - 1 social ties to minimise social interactions (lowest cost) or a fully connected clique with E = N ( N - 1 ) / 2 ties (highest cost).
Evolutionary arguments support that social groups specialise and optimise social interactions to save resources while keeping or increasing the group efficiency 9 – 11 , as for example in response to predators (ecological conditions) 12 or to fitness 13 , 14 . There is also the argument that human social networks have an optimal size to optimise information transfer within groups 15 . Research on urban systems shows that human societies also organise in groups (e.g. cities) to optimise resources like infra-structure and to increase intellectual, social and economic outputs 16 , 17 . These observations lead us to hypothesise that across species and social contexts, the number of social contacts E scales with group size N as E = C N β , where C and β are positive constants.
Until recently, measuring social interactions was laborious. Past research relied on observations of animal and human behaviour or self-reporting of social contacts through questionnaires 4 . A natural limitation of these techniques is the size of the observed populations and potential recalling errors, as for example the inability to accurately identify or quantify each interaction 1 , 4 . Electronic devices (e.g. mobile phones 18 or proximity sensors 19 , 20 ) and online platforms now provide means for passive and accurate recording of spatio-temporal location, communication between animals and between humans, among other forms of animal or human interactions. State-of-the-art electronic data collection is scalable but its ability to detect authentic social interactions may be questioned and should be treated cautiously 21 , 22 .
We collate extensive data to show empirically that the number of social contacts scales super-linearly (i.e. β > 1 ) with group size and that social interactions can be categorised in different exponents β independently of the animal species. We provide evidence that this scaling is necessary to maintain fundamental complex network structures irrespective of existing group sizes. We also fit our data to a social network model and show that a multi-layer structure and the cost of crossing social layers may explain the estimated scaling exponents.
The data sets were collated using online databases of animal and human social networks previously analysed by other authors. All networks were reviewed for consistency and the data sets were standardised such that only unique pairs of social contacts were counted, i.e. self-loops, weighting, timings of contacts, and directions were removed. Social interactions were identified and labelled in the original studies by domain experts via direct observation (animal interactions), questionnaires (offline friendship), electronic devices (spatial proximity), and online platforms (online friendship) (see SI ). To minimise potential ambiguities, each network was constructed based on the specific definition of social interaction in the respective original study. Table 1 shows the number of networks for each type of social interaction and animal class, including captive and free-ranging animals. The network size varies across species and social interactions because of experimental settings, characteristics and limitations of the study populations, e.g. the observation capacity of researchers, cost of technical devices, free-range vs. confined animals, online platforms, or animals living in small groups (see SI ).
Number of networks for each type of social interaction and animal class.
In a total of 611 networks, there are 179 cases of human and 432 cases of non-human social interactions, including 281 captive and 151 free-ranging animals.
Scaling of social interactions
The networks of social interactions were grouped in categories following the type of social interactions as reported in the original studies (Table 1 ). Figure 1 shows the scaling between the number of social contacts E and size N (i.e. the number of interacting individuals) for each of the 6 original categories. We assume that the scaling of social relations is independent of species and test our hypothesis E = C N β by fitting a power-law to the data using logarithmic transformed variables to evenly distribute the data points:
The fitting exercise gives super-linear power-law exponents (i.e. β > 1 ) and strong linear correlations ( 0.55 < r < 0.99 ) for all categories of social interactions (Table 2 ). Assuming a small error ϵ in β ^ , the exponents follow the general law ( β = 1 + a / 4 ) with a ≈ 1 for online friendship ( ϵ = 12 % ), a ≈ 2 for spatial proximity ( ϵ = 4 % ), a ≈ 3 for group membership ( ϵ = 2.6 % ) and offline friendship ( ϵ = 5 % ), and a ≈ 4 for physical contacts ( ϵ = 1 % ) and grooming ( ϵ = 6 % ), despite differences in species and sample sizes (Fig. 1 ).

The number of social connections E versus the group size N across species. Empirical data (each symbol corresponds to a different species) and regression curves (dashed lines) for all 6 categories of social interactions: ( A ) physical contact ( β ^ A = 2.01 , 95 % CI [1.98, 2.04], n = 250 ); ( B ) grooming ( β ^ B = 1.94 , 95 % CI [1.53, 2.36], n = 23 ); ( C ) group membership ( β ^ C = 1.73 , 95 % CI [1.47, 1.99], n = 16 ); ( D ) spatial proximity ( β ^ D = 1.52 , 95 % CI [1.45, 1.59], n = 231 ), with 38 % human and 62 % non-human networks; ( E ) offline friendship ( β ^ E = 1.79 , 95 % CI [1.30, 2.29], n = 67 ); ( F ) online friendship ( β ^ F = 1.22 , 95 % CI [1.06, 1.37], n = 24 ). Details of the fitting in Table 2 . All axes are in log-scale.
Best fitting exponents for the 6 types of social interactions.
The variable n gives for each type of social interaction the number of different networks that was included in the fit. Orthogonal regression is used to account for measurement errors in both axes.
This super-linear scaling indicates increasing densification of social contacts, that is, larger social groups have on average more social contacts per-capita than the smaller ones. It is not surprising that β > 1 because the number of social connections must scale at least linearly with group size ( E ∝ N ) to maintain the social network connected; this is known as the percolation threshold in random networks 23 . If E ≈ N , small perturbations may fragment the network, breaking down the group structure. Furthermore, β > 1 suggests that a super-linear number of contacts are necessary to create and maintain the complex social network structures for the groups to function cost-efficiently irrespective of size.
Social network structure
We study the network structures for each of the six types of social interactions (see “ Methods ”). The clustering coefficient ⟨ c c ⟩ is a local measure of the level of sociality between common contacts of a focal individual (i.e. the fraction of social triangles). Its intensity indicates an evolutionary group advantage as for example fitness benefits 24 , 25 . Networks with higher clustering are relatively more robust since the deletion of a social connection would not significantly affect interaction and communication among close contacts. In our social networks, ⟨ c c ⟩ is constant for varying network size for all types of social contacts (Fig. 2 ). In random networks, the clustering coefficient decays with increasing network size as ⟨ c c ⟩ = ⟨ k ⟩ / N , where ⟨ k ⟩ is the average number of contacts (or edges) in the network 23 . The inset of Fig. 2 F shows the results for the randomised versions of the same online friendship networks (see SI for the other categories). In all categories, there is a higher clustering coefficient than expected on the basis of randomised social contacts (see caption Fig. 2 ). Since the average degree is defined as ⟨ k ⟩ = 2 E / N , we have ⟨ c c ⟩ = ⟨ k ⟩ / N = 2 E / N 2 and thus would need E ∝ N 2 to have constant clustering in random networks. Evolutionary theory implies that more complex structures may emerge in such social systems to optimise resources, e.g. to reap the fitness related benefits, and thus relatively less social contacts become necessary to reach the same level of clustering across group sizes 24 , 25 . For example, for some classes of random heterogeneous networks, ⟨ c c h ⟩ = A / N , where the proportionality constant A depends on the heterogeneity of the distribution of contacts among individuals and is lower than ⟨ k ⟩ 23 .

Network clustering structures. The average clustering coefficient ⟨ c c ⟩ between close contacts vs. network size for ( A ) physical contact (median values for the empirical M emp = 0.94 and randomised M rand = 0.88 versions of the same networks); ( B ) grooming ( M emp = 0.68 and M rand = 0.56 ); ( C ) group membership ( M emp = 0.79 and M rand = 0.69 ); ( D ) spatial proximity ( M emp = 0.50 and M rand = 0.22 ); ( E ) offline friendship ( M emp = 0.37 and M rand = 0.07 ); ( F ) online friendship ( M emp = 0.04 and M rand = 3.5 · 10 - 5 ); the inset is the distribution for the random version of the same networks. Dashed horizontal lines are the median values of the empirical networks. Log-binned (x-axes) Tukey box plots with diamonds representing outliers.
The average length of the shortest-paths ⟨ l ⟩ measures the average distance between any pairs of individuals in the social network and quantifies the communication potential between parts of the network 26 . Shorter average distances (i.e. ⟨ l ⟩ ≪ N , resulting in the small-world effect 27 ) indicate that information flows quickly over the network, which is a fundamental characteristic of efficient group organisation 28 . For physical contacts, grooming, and group membership, ⟨ l ⟩ is constant and slightly higher than one (Fig. 2 A–C). For spatial proximity and offline friendship, the values increase with size following quantitatively similar trends (Fig. 2 D,E). The results for online friendship suggest a constant trend (Fig. 2 F). In all cases, the average path-length is ⟨ l ⟩ < 6 , which is the small-world horizon observed empirically 23 . For all 6 categories, the random versions of the same networks give constant relations albeit generally with lower values (see SI ). In theoretical random networks, the average distance increases slowly with the network size as ⟨ l ⟩ ≈ log ( N ) / log ( ⟨ k ⟩ ) 23 . Nevertheless, the average path-length ⟨ l ⟩ is approximately constant across group sizes if E ∝ N β for β > 1 , since in this case ⟨ l ⟩ ≈ log ( N ) / log ( 2 N β - 1 ) ≈ 1 / ( β - 1 ) . Smaller β thus leads to higher ⟨ l ⟩ , as observed in the analysed networks. In some classes of heterogeneous random networks, ⟨ l ⟩ is also nearly constant with network size 23 . The density of contacts explains the low ⟨ l ⟩ for physical contact, grooming and group membership. The discrepancy of spatial proximity, offline and online friendship with the random case indicates that more complex network structures are being formed in larger groups for these types of social interactions. In sparse networks, like those, a high level of local clustering increases the distance between random pairs of network nodes because of local spots of connectivity redundancy 23 . Taken together, the constant clustering across network sizes (Fig. 2 ) implies that the average distance will necessarily increase (Fig. 3 ), unless followed by a sufficient increase in the number of connections (to maintain low average distances as the group increases). The growth in offline friendship followed by a seemingly constant pattern for online friendship (which has larger sizes) suggests a potential saturation in ⟨ l ⟩ for human friendship in line with the small-world horizon observed in previous studies 23 . Although communication remains efficient (because ⟨ l ⟩ ≪ N ), the benefits of forming larger groups do not compensate the costs of optimising certain network structures, as is the case for other types of social interactions involving physical contact.

Network path structures. The average shortest path-length ⟨ l ⟩ vs. network size in the networks of ( A ) physical contact (median values for the empirical M emp = 1.10 and randomised M rand = 1.05 versions of the original network); ( B ) grooming ( M emp = 1.39 and M rand = 1.29 ); ( C ) group membership ( M emp = 1.30 and M rand = 1.09 ); ( D ) spatial proximity ( M emp = 2.16 and M rand = 2.00 ); ( E ) offline friendship ( M emp = 3.19 and M rand = 3.36 ); ( F ) online friendship ( M emp = 4.66 and M rand = 4.97 ). Dashed horizontal lines are the median values of the empirical networks. Log-binned (x-axes) Tukey box plots with diamonds representing outliers.
Multi-layer model
Multi-layer models can be used to represent the underlying generative mechanisms through which individuals combine skills and affinity to build up more complex social groups. From single individuals to the entire population, individuals may be stratified in layers (or levels) corresponding to different groups 29 . For example, living in households (layer 1) within neighbourhoods (layer 2) that in turn are part of cities (layer 3), and so on, seems natural for humans. While people mostly interact with those in the same group (e.g. within the same household), interactions across groups are less frequent 30 (e.g. between different households in the same neighbourhood). Interactions across groups at the same layer are necessary to define higher-order groups, i.e. a group at the next higher layer, as for example a neighbourhood is a result of interactions between individuals from different households. Multi-layer models have been used to explain spatial relations in vascular 31 and infrastructure 17 systems. We argue that such models are also of value for social groups, not necessarily spatially bound, since multi-layer organisation has been observed across animal species in which a relation between group sizes in different layers vary from nearly 2.5 in primates to about 3 for other mammals including humans 14 , 32 . This means that individuals are organised as multiples of 3, for example, in groups of 5 (layer 1), 15 (layer 2), 45 (layer 3), and so on. The model detailed below does not aim to reproduce all structures of the 611 analysed networks but focuses on the scaling exponents β .
The self-similar multi-layer group structure is mathematically represented as a branching tree with a group at layer h split into b sub-groups at layer h - 1 (Fig. 4 ). At the highest layer h max , all individuals belong to a single social group, i.e. N = b h max , and at the lowest layer ( h min = 0 ), each group is formed by a single unique individual. In this model, individuals i and j make a social contact ( i , j ) with probability p Δ h ( i , j ) dependent on the distance Δ h between the layers that separate them. Closer individuals (e.g. at distance Δ h = 1 because they are living in the same neighbourhood or belonging to the same social group) are more likely to interact than individuals living far apart (e.g. at distance Δ h = 2 because they are living in different cities or belonging to different social groups), i.e. p Δ h ( i , j ) decreases with Δ h . The multi-layer tree-like structure only defines the distance Δ h between the groups that is in turn used to form contacts in the social network (Fig. 4 ); the resulting social network only has tree-like structure for sparse networks, i.e. when E ≪ N 2 . The self-similarity between layers implies that p Δ h ( i , j ) / p Δ h - 1 ( i , j ) = c o n s t 33 . A power-law of the form p Δ h ∝ c - Δ h , with c > 1 , satisfies this relationship. The parameter c represents the cost to make social interactions across layers, that we assume is lower than the cost to create a new layer, i.e. c < b , because multiple contacts are necessary to establish a new layer. For a given individual i , the expected number of social connections ⟨ e ⟩ i is:
For 1 ≤ c < b , the sum converges:
Since N = b h max and c h max = b h max log b ( c ) , we get:
Therefore, the total number of social connections is:
The multi-layer model implies that β = 2 - log b ( c ) . Assuming that b = 2.5 14 , the cost of connections is thus c A = 1 for physical contact ( β A ≈ 2 ), c B = 1 for grooming ( β B ≈ 2 ), c C = 1.26 for group membership ( β C ≈ 1.75 ), c D = 1.58 for spatial proximity ( β D ≈ 1.5 ), c E = 1.26 for offline friendship ( β E ≈ 1.75 ) and c F = 1.99 for online friendship ( β F ≈ 1.25 ). This cost is associated to crossing (virtual) barriers between social groups that might cause the creation of larger groups. The low cost ( c = 1 ) for physical contact and grooming means that p Δ h = 1 , i.e. the probability to form connections is independent of the social distance Δ h , collapsing the assumption of multi-layer structure. For such types of social interactions, the connections within the same social group are favoured; individuals do not groom in different social groups nor make persistent physical contacts, except physical contacts for conflict that would not be reflected in our data. This effect is related to the high clustering coefficient reported in previous sections and may explain the relatively small size of such networks. The number of social contacts for such activities is limited within the same social group.

Multi-layer social model. The underlying multi-layer structure (left) defines the probability p Δ h ( i , j ) ∝ c - Δ h of forming connections between individuals i and j in the social network (right). If c = 1 , everyone interacts with everyone else leading to a fully connected network whereas for higher c , interactions between closer individuals (lower h ) are more common. For example, the distance Δ h A , B = 1 and Δ h A , D = 2 . With cost c = 1 , edges ( A , B ) and ( A , D ) are equally likely ( p 1 = p 2 ∝ 1 ), whereas with higher cost, e.g. c = 2 , edge ( A , B ) is more likely ( p 1 ∝ 1 / 2 ) to occur than edge ( A , D ) ( p 2 ∝ 1 / 4 ).
Online friendship, on the other hand, is costly ( c ≈ 1.99 ) in terms of crossing social boundaries to connect individuals from different social groups 34 , e.g. with different tastes, ideas, location, age, and so on. Socially closer individuals would be favoured here as well since it is harder to be friends with dissimilar people than with those similar to each other 30 . However, given that online connections are cheap to establish and maintain (i.e. do not need nurturing and resources), the multi-layer structure becomes relevant with a non-negligible number of socially distant connections being formed. Furthermore, online friendship typically mixes (real) friends, acquaintances, relatives, and co-workers, each belonging to different social groups, with some individuals acting as social brokers. For example, online friendship is more easily established between those studying in the same school than at different schools; however, inter-school friendship is facilitated by the online platform, though socially costly (lack of face-to-face interactions, no common friends, building trust). For networks derived from mobile phone communication in urban populations, a scaling exponent β = 1.15 has been reported 35 – 37 . Such mobile communication data sets mix professional and personal relations which possibly also leads to higher costs in the sense of crossing social boundaries. In one study, a constant clustering coefficient has been also observed suggesting that similar underlying principles may explain the formation of such social or communication structures 37 . The multi-layer structure becomes less relevant for offline friendship ( c ≈ 1.32 ) that are typically more spatially constrained in our data. For example, students or prison inmates will report friendship with those around them. In schools, from where most of our data come from, the social structure is seen at the class and school layers only. Given experimental limitations, it is often not possible to report friends outside the study setting, which could reveal higher social layers, e.g. neighbourhood friends. It is possible that the exponent β for friendship is thus between what we estimated for offline and online friendship if all layers of friends and not only those in the same study setting were reported.
Our analysis finds an intermediate exponent ( β = 1.5 ) and cost ( c ≈ 1.58 ) for spatial proximity. Spatial proximity is a particular type of social interaction. Grooming, physical contact and human friendship are well-defined interactions identified, respectively, by observing joint activities or by directly inquiring individuals. However, spatial proximity interactions are measured by sensors or direct observation and capture a mixture of social situations. Spatial proximity might reflect affinity, trust and friendship between individuals and animals sharing the same space 30 , e.g. persistent spatial proximity between pairs of cows 38 , or behavioural or trait similarity, i.e. homophily, as for example friends visiting a museum 39 or health-care workers in hospitals 40 . On the other hand, spatial proximity interactions might simply reflect spatial constrains forcing individuals and animals to be in close proximity during periods of time, e.g. a group of visitors of an art exhibition 39 or confined animals 38 . Nevertheless, also in the later, affinity and trust are reflected in the proximity contacts. As discussed above, it is possible that friendship at the society layer likely follows patterns intermediate to those observed in the online ( β = 1.25 ) and offline ( β = 1.75 ) categories. The existing literature associating friendship to time that individuals spent together 30 and the observation that spatial proximity contacts follow an intermediate exponent ( β = 1.5 ) suggest a potential link between these social interactions. We cannot make a strong association between the two types of social interactions due to lack of data of offline friendship in larger populations. Previous modelling exercises in urban populations suggest that β B = 1.5 can be explained by mobility ( H = 2 , where H is the Hausdorff dimension of a path in space) over two dimensional ( D = 2 ) spaces based on the assumption that fully-mixed populations may fully explore a given area 17 . While this assumption may hold within, e.g. schools, museums or barns, it does not apply on larger spatial areas since humans and animals are territorial and tend to spend most of time within certain locations 41 or with certain individuals 30 . On the other hand, the same model suggests that contacts per-capita scale as 0.25 (i.e. β = 1.25 ) under the same conditions (i.e. H = D = 2 ). This fits well to our findings for offline friendship, where people may virtually explore the whole social space and potentially interact with different individuals.
Conclusions
Our findings reveal key aspects of the organisation of animal social networks. Though primates and non-primates (including humans) are more represented than other animals in our data set, the universal scaling relations E = C N β between the number of social contacts E and size N suggest common organisation principles across animal species that can be explained by multi-layer models designed to maintain the functioning of the social groups 14 , 32 . Different scaling exponents following the general relation β = 1 + a / 4 , with a ≈ 1 , 2 , 3 , 4 allow us to distinguish types of social interactions and to infer network structures underlying those interactions. For all types of social interactions, the local clustering remains constant for increasing network sizes albeit having different intensity in each case. Physical contacts, grooming and group membership have similar constant median values that are higher than observed for spatial proximity, offline and online friendships. The average path-length is also constant and follow the small-world pattern (i.e. ⟨ l ⟩ ≪ N ) for most cases with the exception of spatial proximity and offline friendship where a quantitatively similar positive trend is observed with values below the small-world horizon of ⟨ l ⟩ ≈ 6 previously observed in social networks 23 .
One may argue that humans differ from other animals by developing more efficient social network structures, with relatively less contacts for larger network sizes, and thus lowering the scaling exponents. There is a quantifiable relationship with brain and group sizes, along with the complexity of the interactions. Humans are able to process the cognitive demand of other forms of relationships such as friendship, rather than mating and dominance relations that often occur within other animals and species 42 . The common scaling pattern observed across species and particularly for spatial proximity weakens the hypothesis that animals differ. Our results suggest that the type of social interaction, and to a lesser extent, the group size, are more relevant to determine the scaling exponents than the animal species. We reached this conclusion by combining data from different species. More statistical power could be achieved with a larger sample of network data for specific combinations of social interactions and species in order to study these relations separately. Given the multi-layer structure of social networks and experimental constraints, offline friendship data sets are limited to relatively small social circles 30 . If one could map higher social layers, the scaling exponent could decrease, likely to the same value as observed for spatial proximity. If this is confirmed in future studies, we will be able to infer that spatial proximity is a proxy of friendship across animals species 30 .
Physical contact, grooming and group membership are associated with more robust and topologically efficient networks (since clustering is higher and path-lengths are shorter) than friendship and proximity interactions. This social cohesion is a result of homophily and coordination to maintain group functioning, which likely creates smaller groups in these categories relative to friendship and proximity categories because of the cost of nurturing contacts. The frequency and number of social interactions leading to stable social contacts are also important to regulate diffusion processes such as communication 26 , innovation 16 , 17 , infectious diseases 19 , 43 and social phenomena 30 , 44 . Our results suggest that physical contacts and grooming are more efficient than proximity to facilitate spread phenomena at the population (network) level. Online friendships are associated to looser social structures easier to fragment as the groups increase in size. The relatively high cost of nurturing too many online social contacts across social layers restrains the opportunities to generate higher clustering or common friends, and create redundant structures, as observed in the smaller networks related to activities necessary to keep the group functioning.
Although we focus on temporally stable social networks 45 , the availability of temporal information and intensity of certain social interactions could also help to understand the formation and dissolution of social contacts and how particular network structures are formed. Future research should add a quality measure to social interactions (e.g. via weights or temporal dynamics) to investigate the varying importance of creating and maintaining particular structures 46 . Strong super-linear scaling implies prohibitive social costs to maintain larger groups for some types of social interactions. The questions on whether there is a maximum or optimal group size in which efficient groups can exist and fitness is maximised 47 , or whether more complex network structures are necessary to sustain larger groups, remain open.
The data sets used in this study were collected using public network data repositories. A list of repositories and a full list of the original references for the 611 data sets are available in the SI . The 6 types of social interactions: physical contact, grooming, group membership, spatial proximity, offline friendship and online friendship were identified and labelled in the original studies by domain experts via direct observation (animal interactions), questionnaires (offline friendship), electronic devices (spatial proximity), and online platforms (online friendship). All 611 networks were standardised for the analysis, including the removal of self-loops, edge directions, and edge weights.
A network G of size N is defined as a set of N nodes i and a set of E edges ( i , j ) connecting nodes i and j . A node represents either a person or an animal. An edge represents a social connection of a specific type. In an undirected network, edges are reciprocal, i.e. ( i , j ) = ( j , i ) . In a network without self-loops, there is no edge ( i , i ).
The clustering coefficient of a node i is given by:
where e i is the number of edges (connections) between the n i nodes directly connected to node i . The average clustering coefficient of the network G is thus:
The topological distance between the nodes i and j is the length of the shortest-path l ij in number of edges. It is calculated within the largest connected component of the network G . In the largest connected component, there is at least one path between any pairs of nodes i and j . The average shortest-path length is:
Supplementary Information
Acknowledgements.
The authors thank Luana de Freitas Nascimento for helpful discussions.
Author contributions
L.R. designed the research, made the analysis and wrote the draft; J.R., K.S., M.S. contributed with methods; All authors revised the manuscript.
Competing interests
The authors declare no competing interests.
Publisher's note
Springer Nature remains neutral with regard to jurisdictional claims in published maps and institutional affiliations.
The online version contains supplementary material available at 10.1038/s41598-021-92025-1.
Animal Groups Journeys
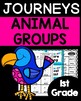
Description
Questions & answers, teaching second grade.
- We're hiring
- Help & FAQ
- Privacy policy
- Student privacy
- Terms of service
- Tell us what you think
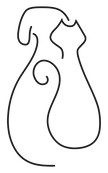
Humane Society of the Palouse
"helping those who cannot help themselves.", save a pet,, donate now.

Roughly 3 years old.
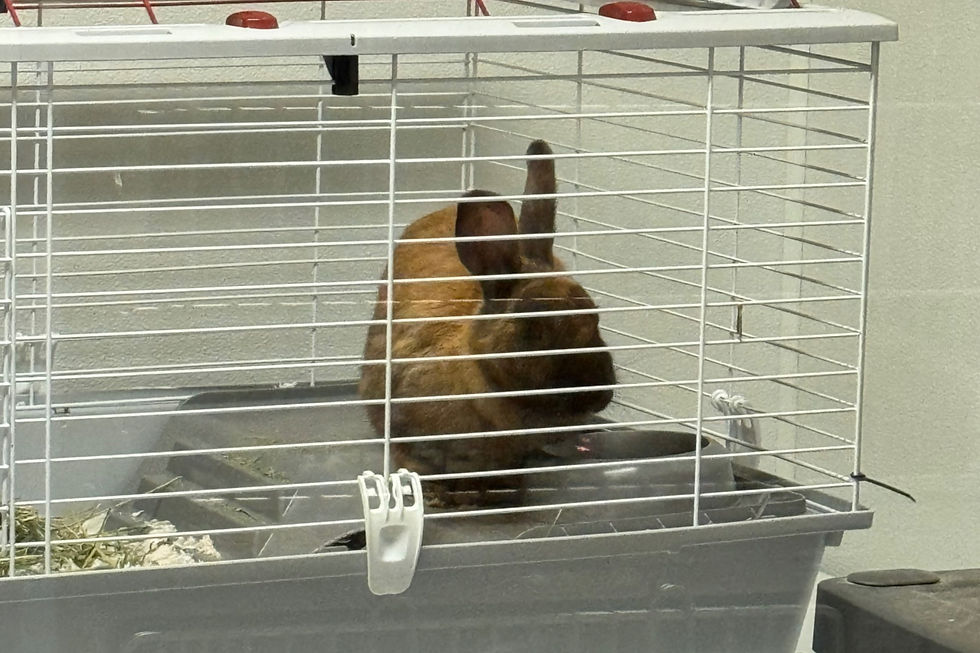
Female rabbit.
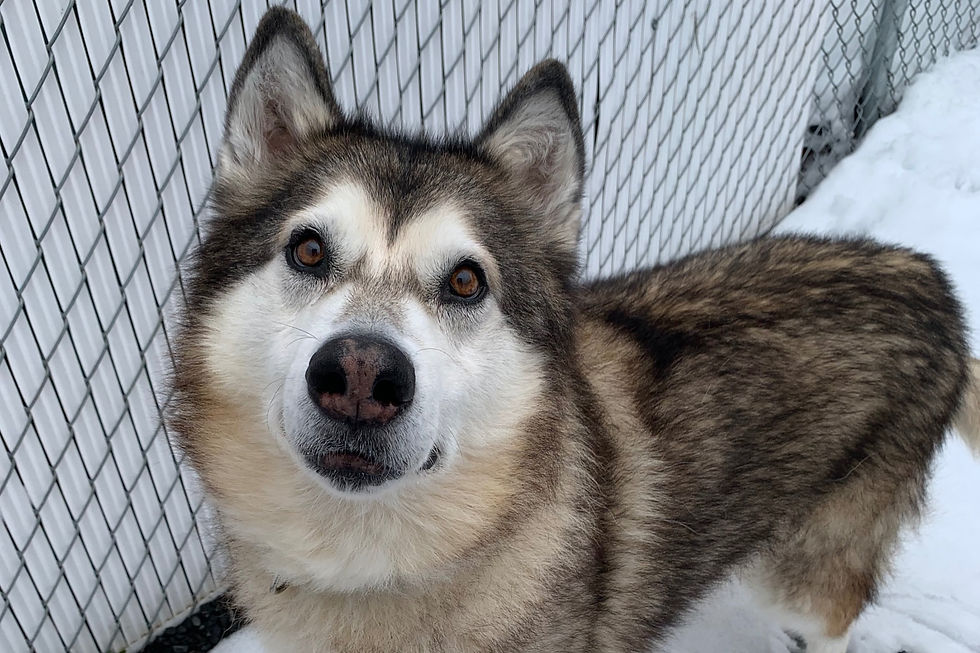
Roughly 9 years old.
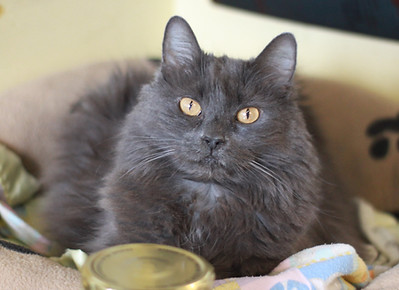
Wobbles, forever the queen of HSoP ♥
The Five Freedoms
Hsop is dedicated to providing the animals in our facility with the highest level of care possible during their stay with us. adhering to the five freedoms ensures we are giving the companion animals in our care everything they need to live a healthy and happy life. staff monitors each pet at hsop individually to identify their specific mental and physical needs, so we can be sure to give them the most humane treatment during their temporary stay with us. our shelter is committed to providing animals with:.
1. Freedom from Hunger and Thirst
An adequate and healthful diet, with ready access to fresh drinking water.
2. Freedom from Discomfort
An appropriate living environment including a clean and comfortable resting area.
3. Freedom from Pain, Injury, and Disease
Prevention or rapid diagnosis, and treatment for injury or illness.
4. Freedom to Express Normal Behaviors
Sufficient space, exercise, and ability to be with animal's own kind.
5. Freedom from Fear and Distress
Conditions and treatment that avoid mental suffering and stress.
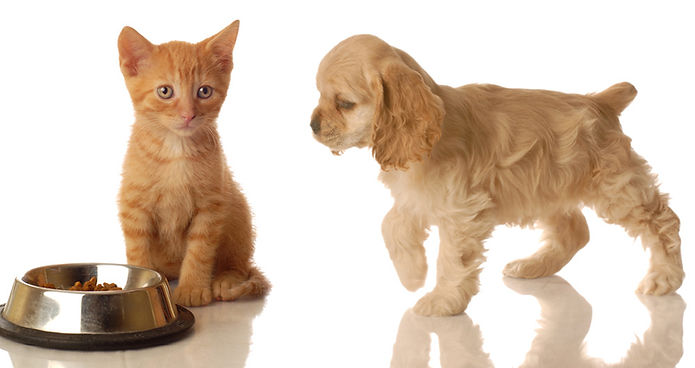
Enrichment Program
Help us keep our pets happy!
Here at HSoP we strive to ensure our animals receive the highest level of care possible. This includes physical, mental, and emotional stimulation while they stay with us. We do our best to make sure each animal in our facility receives all preventative and necessary medical attention, as well as enrichment in their kennels to keep them entertained. Did you know animals can go stir crazy when they are confined in a small space for periods of time with no distractions? This is a common issue for animal shelters nationwide, and we are determined to limit that likelihood as much as possible for the animals here at HSoP.
The staff here at HSoP are dedicated to making sure each animal has plenty of stimulation both physical and mental, to keep them from getting bored. Our wonderful volunteers help with this by taking the animals out of their kennels for some free time in a different area.

Thank you Nylabone for donating roughly 250 Chews!
Our dogs enjoy going to their outside kennels and side yard to play fetch, stretch their legs, and change their scenery! Our cats love to get out of their kennels and go into our adoption room so they can stretch their legs, chase some toys, and get some extra special cuddles!
We want our animals to have a great experience here while they wait for their new homes, so we are starting an Enrichment Program. This program will be dedicated to finding new and innovative ways to keep our furry friends busy and happy in their kennels!

To achieve this goal of ours, we need your help! We have created a Wishlist on our amazon of all the items we think could be of great use for this program. We hope you will take a moment to browse through our list and pick a few thin gs you would like to donate to our furry friends here at HSoP!
https://a.co/1SSv51R
Donations can be mailed to 2019 E. White Ave. Moscow, ID 83843
Thank you Starmark for donating 25 treat dispensing chew balls!
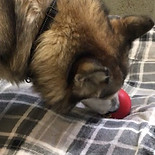
Thank you Petfinder Foundation for your grant of 15 Kong Toys to benefit our canine friends of HSoP. With this grant we are able to provide our dogs with both mental and physical stimulation. Keeping our animals happy and healthy during their stay with us is our number one priority. With donations and grants like this, we can continue to give our animals the highest quality of care possible.

IMAGES
VIDEO
COMMENTS
Listen along as elementary teacher Mrs. Morris does an engaging read of "Animal Groups" from the Journey's series, first grade lesson 15. Check out my merch...
This is a read aloud of the story, Animal Groups. Students can follow along in their anthologies, or with the highlighted words on the screen! Enjoy! :)#Anim...
AnimalsWords to KnowRead and ComprehendCompare and contrastInformational textDig DeeperYour turnAnimal picnicPlayCompare TextsGrammarInformative WritingReport
Animal Groups Journeys Unit 3 Lesson 15 First Grade Supplement Activities Common Core aligned Created and tested by a first grade teacher. Pg. 3 Spelling Nests! -unscramble the spelling words on each nest, cut out the owl boxes, match the owls with the correct spelling words to the nests and glue them Pg. 4-5 Rainbow Penguins! - color the ...
Animal Groups Supplement Activities Journeys 1st Grade Unit 3 Lesson 15 **Focus wall posters in this pack. They are in the zip file in their own pdf. Guess The Word p.5 Color Me By Words to know/ Spelling p.6-7 Roll a Spelling Word p.8-10 Vocab Flash Cards p.11-12 Word Team-Up p.13-14 Compare and Contrast Animal Groups Booklet p.15-19 -er and ...
feathers. what covers a bird's body. group. a number of living things that are alike in some way. hair. thin strands that grow from the skin of humans and other animals. mammals. warm-blooded animals with hair or fur that make milk. reptiles.
Animal Groups Journeys Unit 3 Lesson 15 First Grade Supplement Activities Common Core aligned Created and tested by a first grade teacher. Pg. 3 Spelling Nests! -unscramble the spelling words on each nest, cut out the owl boxes, match the owls with the correct spelling words to the nests and glue them Pg. 4-5 Rainbow Penguins! - color the ...
Animal Groups Journeys Lesson 15, Unit 3. There are 100 pages included in this story unit! I am currently working on all First Grade Journeys and revising all of the the ones that I have previously made.
Madison County Schools / Overview
We used these models to study 7,009 species belonging to 56 groups of tetrapods. What we found For 91% of the animal groups we studied, models that included species characteristics were better ...
Animal Groups. Explore this website to learn more after reading Animal Groups by James Bruchac. Meet the Author. Powered by Create your own unique website with customizable templates. Get Started. Home About the Author Animal Groups ...
Journeys Lesson 15 | Animal Groups quiz for 1st grade students. Find other quizzes for Other and more on Quizizz for free!
3554 results for 'journeys animal groups'. Animal Groups Sort Group sort. by Rcrull. G1 G2 G3 G4 Science animal groups animals life science. Journeys Lesson 16: Mr. Tanen's Tie Trouble Vocabulary Quiz. by Missyun. G2 Journeys Reading. Yonder Mountain Vocabulary Random wheel. by Lisaevans.
Animal Groups Journeys quiz for 1st grade students. Find other quizzes for English and more on Quizizz for free!
Lesson 15: Animal Groups by James Bruchac Question to think about: How are animals the same and different? Words to Know: bird, both, eyes, fly, long, or, those, and walk Spelling Words: time, like, kite, bike, white, and drive practice here Phonics: Using kn, wr, gn, mb, and long i (CVCe); Using -ine, -ite Comprehension: Compare/contrast and monitor/clarify
About Press Copyright Contact us Creators Advertise Developers Terms Privacy Policy & Safety How YouTube works Test new features NFL Sunday Ticket Press Copyright ...
Guess The Word p.5 Color Me By Words to know/ Spelling p.6-7 Roll a Spelling Word p.8-10 Vocab Flash Cards p.11-12 Word Team-Up p.13-14 Compare and Contrast Animal Groups Booklet p.15-19 -er and -est Animal Heads p.20-26 The Verb Be foldable Booklet p.27 Animal Groups Tri-Fold p.28-29 Spelling/ Words to Know Writing practice p.30-31 Thanks ...
This packet includes activities to go along with the skills being taught with the story "Animal Groups" in the Journeys® reading series. This packet includes the following activities: Long i Match Up {picture to word}-ine and -ite Word Sort Build words with -ine {-ine word family} Build words with -ite {-ite word family} Ghost Letter Digraph Anchor Chart idea Ghost Letter Digraph Sort {wr ...
We collate 611 animal social networks and show that the number of social contacts E scales with group size N as a super-linear power-law E = CNβ for various species of animals, including humans, other mammals and non-mammals. We identify that the power-law exponent β varies according to the social function of the interactions as β = 1 + a/4 ...
Laika (/ ˈ l aɪ k ə / LY-kə; Russian: Лайка, IPA:; c. 1954 - 3 November 1957) was a Soviet space dog who was one of the first animals in space and the first to orbit the Earth. A stray mongrel from the streets of Moscow, she flew aboard the Sputnik 2 spacecraft, launched into low orbit on 3 November 1957. As the technology to de-orbit had not yet been developed, Laika's survival was ...
4.9. (153) $4.00. PDF. This set includes worksheets to supplement your Journeys curriculum for first grade. This file goes with Unit 3 Lesson 15: Animal Groups.It includes:5 phonics worksheets (i with silent e; a and i with silent e; silent letters mb, gn, wr, kn) with answer keys5 comprehension worksheets (comparing and contrasting animal ...
Animal Groups Journeys Lesson 15, Unit 3 There are 100 pages included in this story unit! I am currently working on all First Grade Journeys and revising all of the the ones that I have previously made. Please always check back and look under "My Purchases". If there have been any changes, it...
Keeping our animals happy and healthy during their stay with us is our number one priority. With donations and grants like this, we can continue to give our animals the highest quality of care possible. The mission of Humane Society of the Palouse is to ensure the humane treatment, welfare, and safety of companion animals in Moscow and Latah ...
About Press Copyright Contact us Creators Advertise Developers Terms Privacy Policy & Safety How YouTube works Test new features NFL Sunday Ticket Press Copyright ...
Five starving dolphins were rescued from an abandoned amusement park in the Bahamas, following the deaths of eight others, an animal welfare group said. The park, located on Blackbeard Cay, once ...